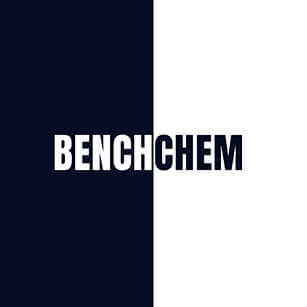
FERROCENEACETIC ACID
- Click on QUICK INQUIRY to receive a quote from our team of experts.
- With the quality product at a COMPETITIVE price, you can focus more on your research.
Overview
Description
Ferroceneacetic acid is an organometallic compound with the chemical formula C12H12FeO2 It consists of a ferrocene moiety attached to an acetic acid group Ferrocene itself is a well-known sandwich compound where an iron atom is sandwiched between two cyclopentadienyl rings
Mechanism of Action
Target of Action
Ferroceneacetic acid (FAA) is a versatile catalyst that has been used in various applications . Its primary targets include the Escherichia coli ribonucleotide reductase subunit R2 and cancer cells . The ribonucleotide reductase subunit R2 plays a crucial role in DNA synthesis and repair, while cancer cells are the primary targets in antitumor applications .
Mode of Action
FAA interacts with its targets through various mechanisms. It acts as a reducing agent for the reduction of the Escherichia coli ribonucleotide reductase subunit R2 . In cancer cells, FAA acts as an antitumor agent by generating active oxygen species, which induce oxidative DNA damage .
Biochemical Pathways
FAA affects several biochemical pathways. It plays a role in the hydroxylation and polymerization reactions . In cancer cells, FAA induces the generation of reactive oxygen species (ROS), leading to oxidative DNA damage . This process is part of the ferroptosis pathway, a form of programmed cell death that is iron-dependent and caused by increased cellular ROS and lipid peroxidation .
Pharmacokinetics
Its molecular weight (24407) and form (powder) suggest that it may have good bioavailability
Result of Action
The molecular and cellular effects of FAA’s action are significant. As an antitumor agent, FAA induces oxidative DNA damage in cancer cells, leading to cell death . This is achieved through the generation of active oxygen species . In addition, FAA has been used in the preparation of silver nanoparticles and in the synthesis of redox-triggered drug delivery vesicles for cancer cell treatment .
Action Environment
The action of FAA can be influenced by various environmental factors. For instance, the electrochemical properties of FAA have been found to be influenced by several factors including the ferrocene positions in different environments and applied potentials . Moreover, FAA’s stability and redox properties make it suitable for use in various conditions, including those that might be present in biological systems .
Biochemical Analysis
Biochemical Properties
Ferroceneacetic acid plays a significant role in biochemical reactions. It interacts with various enzymes, proteins, and other biomolecules. For instance, ferrocene derivatives have been synthesized by the Suzuki cross-coupling reaction of ferroceneboronic acid with various aryl/heteroaryl halides . The derivatives have been characterized by 1H NMR, 13C NMR, IR, HRMS, and elemental analysis .
Cellular Effects
This compound has notable effects on various types of cells and cellular processes. It influences cell function, including impacts on cell signaling pathways, gene expression, and cellular metabolism. For example, it has been reported as an antitumor agent via the generation of active oxygen species, which induce oxidative DNA damage in cancer cells .
Molecular Mechanism
At the molecular level, this compound exerts its effects through binding interactions with biomolecules, enzyme inhibition or activation, and changes in gene expression. For instance, when the side group of ferrocenes were an electron-donating, lower oxidation was displayed. On the other hand, the strong electron-withdrawing group had a higher oxidation potential .
Preparation Methods
Synthetic Routes and Reaction Conditions: Ferroceneacetic acid can be synthesized from acetylferrocene through a multi-step process. One common method involves the reaction of acetylferrocene with morpholine, sodium sulfide nonahydrate, and sulfur at 128°C for 7 hours. The reaction mixture is then treated with sodium hydroxide in ethanol and water under reflux for 4 hours. The product is isolated by adjusting the pH with hydrochloric acid, resulting in a yellow-brown solid .
Industrial Production Methods: While specific industrial production methods for this compound are not widely documented, the laboratory synthesis methods can be scaled up for industrial purposes. The key steps involve careful control of reaction conditions and purification processes to ensure high yield and purity.
Types of Reactions:
Oxidation: this compound can undergo oxidation reactions, often facilitated by oxidizing agents such as hydrogen peroxide or potassium permanganate.
Reduction: It can be reduced using reducing agents like sodium borohydride.
Substitution: The acetic acid group can participate in substitution reactions, where the hydroxyl group is replaced by other functional groups.
Common Reagents and Conditions:
Oxidation: Hydrogen peroxide, potassium permanganate.
Reduction: Sodium borohydride.
Substitution: Various nucleophiles under acidic or basic conditions.
Major Products:
Oxidation: Products include ferroceneacetate and other oxidized derivatives.
Reduction: Reduced forms of this compound.
Substitution: Substituted ferrocene derivatives depending on the nucleophile used.
Scientific Research Applications
Ferroceneacetic acid has diverse applications in scientific research:
Comparison with Similar Compounds
Ferrocene: The parent compound, consisting of an iron atom sandwiched between two cyclopentadienyl rings.
Acetylferrocene: A derivative with an acetyl group attached to the ferrocene moiety.
Ferrocenemethanol: A derivative with a hydroxymethyl group attached to the ferrocene moiety.
Uniqueness of Ferroceneacetic Acid: this compound is unique due to the presence of the acetic acid group, which enhances its reactivity and potential applications. This functional group allows for a wider range of chemical reactions and applications compared to its parent compound, ferrocene, and other derivatives.
Properties
CAS No. |
1287-16-7 |
---|---|
Molecular Formula |
C12H12FeO2 10* |
Molecular Weight |
244.07 |
Origin of Product |
United States |
Disclaimer and Information on In-Vitro Research Products
Please be aware that all articles and product information presented on BenchChem are intended solely for informational purposes. The products available for purchase on BenchChem are specifically designed for in-vitro studies, which are conducted outside of living organisms. In-vitro studies, derived from the Latin term "in glass," involve experiments performed in controlled laboratory settings using cells or tissues. It is important to note that these products are not categorized as medicines or drugs, and they have not received approval from the FDA for the prevention, treatment, or cure of any medical condition, ailment, or disease. We must emphasize that any form of bodily introduction of these products into humans or animals is strictly prohibited by law. It is essential to adhere to these guidelines to ensure compliance with legal and ethical standards in research and experimentation.