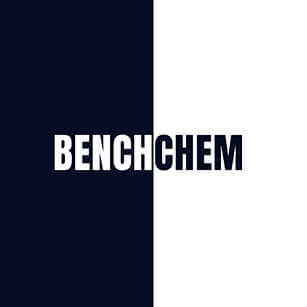
Bromoferrocene
- Click on QUICK INQUIRY to receive a quote from our team of experts.
- With the quality product at a COMPETITIVE price, you can focus more on your research.
Overview
Description
Bromoferrocene (C₁₀H₉BrFe, molecular weight: 264.93 g/mol) is a halogenated derivative of ferrocene, featuring a bromine atom substituted on one cyclopentadienyl (Cp) ring. It appears as a brown-red solid or semi-solid with a melting point of 26–32°C and is insoluble in water . Its CAS registry number is 1273-73-0, and it is commercially available at 97% purity . This compound serves as a critical precursor in organometallic synthesis, enabling the preparation of disubstituted ferrocenes, phosphine ligands, and bioactive molecules . Safety protocols highlight its irritant properties (H315-H319-H335) and recommend handling under inert conditions for air-sensitive reactions .
Preparation Methods
Traditional Lithiation-Bromination Methods
The lithiation-bromination approach remains a foundational method for bromoferrocene synthesis. In a representative procedure, ferrocene (3.72 g, 20.0 mmol) is treated with potassium tert-butoxide (1.615 g, 15.2 mmol) in tetrahydrofuran (THF) at −78°C, followed by dropwise addition of t-BuLi (40.0 mmol) . Subsequent reaction with 1,1,2,2-tetrabromoethane (20.0 mmol) yields crude this compound, which is purified via dichloromethane extraction and magnesium sulfate drying. This method achieves moderate yields (50–60%) but requires stringent temperature control and inert atmospheres to prevent side reactions .
Key Data:
Parameter | Value |
---|---|
Starting Material | Ferrocene |
Lithiating Agent | t-BuLi |
Brominating Agent | 1,1,2,2-Tetrabromoethane |
Solvent | THF |
Yield | 50–60% |
Stannylferrocene Bromination Approach
A scalable route involves stannylferrocene intermediates. For instance, 1,1′-bis-(tri-n-butylstannyl)ferrocene reacts with bromine (2.2 equiv.) in dichloromethane, undergoing a self-indicating titration reaction . The endpoint is visually discernible through color changes from deep green to orange, eliminating the need for chromatographic purification. Organotin by-products are removed via aqueous sodium thiosulfate washes, affording 1,1′-dithis compound in 70–85% yield . This method excels in large-scale production due to minimal by-product interference and straightforward workup.
Optimization Insight:
-
Solvent Choice: Dichloromethane enhances reactivity and solubility of stannyl precursors .
-
Stoichiometry: Excess bromine (2.2 equiv.) ensures complete conversion, avoiding residual stannyl groups .
Microwave-Assisted Synthesis Techniques
Microwave irradiation significantly accelerates this compound synthesis. A patented method employs acetyl ferrocene and N-bromosuccinimide (NBS) in acetonitrile under 750W microwave radiation . The reaction completes within minutes, as monitored by thin-layer chromatography (TLC). Post-reaction, solvent evaporation and petroleum ether washing yield α-bromoacetyl ferrocene with 42–47% efficiency . While rapid, this method necessitates specialized equipment and precise power modulation to prevent decomposition.
Representative Conditions:
Parameter | Value |
---|---|
Reactants | Acetyl ferrocene, NBS |
Solvent | Acetonitrile |
Microwave Power | 750W |
Reaction Time | 5–10 cycles of 5s irradiation |
Yield | 42–47% |
Bromination via Permercurated Ferrocene Intermediates
Polybrominated ferrocenes are accessible through permercurated precursors. Treatment of ‘[C₁₀(HgOAc)₁₀Fe]’ with KBr₃ in aqueous medium generates deca-, nona-, and octathis compound derivatives . X-ray crystallography confirms the structure of [(C₅H₂Br₃)₂Fe], featuring triclinic symmetry (space group P) with lattice parameters a = 7.0903 Å, b = 7.4318 Å, and c = 13.8071 Å . Although academically intriguing, this method’s complexity and low yields (≤30%) limit industrial applicability.
Comparative Analysis of Preparation Methods
The table below juxtaposes critical metrics across synthesis routes:
Method | Yield (%) | Scalability | Equipment Needs | By-Product Handling |
---|---|---|---|---|
Lithiation-Bromination | 50–60 | Moderate | Cryogenic | Complex |
Stannylferrocene Route | 70–85 | High | Standard | Simple |
Microwave-Assisted | 42–47 | Low | Specialized | Moderate |
Permercurated Pathway | ≤30 | Low | Advanced | Challenging |
The stannylferrocene method emerges as optimal for large-scale production, whereas microwave techniques suit rapid, small-batch synthesis .
Challenges and Optimization Strategies in this compound Synthesis
Challenges:
-
Toxicity: Bromine and organotin reagents pose handling risks, necessitating fume hoods and personal protective equipment .
-
By-Product Removal: Mercury and tin residues require meticulous aqueous washes or column chromatography .
Optimizations:
Chemical Reactions Analysis
Types of Reactions
Bromoferrocene undergoes various types of chemical reactions, including:
Substitution Reactions: this compound can participate in nucleophilic substitution reactions where the bromine atom is replaced by other nucleophiles.
Oxidation Reactions: This compound can be oxidized to form ferricenium ions, which are useful intermediates in various chemical processes.
Coupling Reactions: It can also undergo coupling reactions with other organometallic compounds to form more complex structures.
Common Reagents and Conditions
Common reagents used in the reactions of this compound include copper(I) chloride, pyridine, and various oxidizing agents. The reactions are typically carried out under mild conditions, often at room temperature, to ensure high selectivity and yield .
Major Products Formed
The major products formed from the reactions of this compound include chloroferrocene, ferricenium ions, and various coupled products depending on the specific reaction conditions and reagents used .
Scientific Research Applications
Catalytic Applications
Bromoferrocene serves as a crucial precursor for synthesizing various functionalized ferrocene derivatives that act as catalysts in organic reactions. These derivatives are particularly valuable in:
- Olefin Polymerization : Ferrocene-based catalysts have been developed for efficient olefin polymerization processes, enhancing the production of polymers with desirable properties.
- Cross-Coupling Reactions : this compound can participate in cross-coupling reactions, where it is used to form carbon-carbon bonds, essential in synthetic organic chemistry.
Recent studies have demonstrated the effectiveness of this compound in remote catalytic functionalization, where it has been utilized to produce 3-borylated products under specific catalytic conditions . This highlights its potential in developing new catalytic systems that can achieve high yields with selectivity.
Materials Science
The thermal stability and ease of functionalization of this compound make it a promising candidate for creating novel materials. Its applications include:
- Redox Dynamic Polymers : this compound derivatives are incorporated into redox-active polymers that can be utilized in energy storage devices due to their ability to undergo reversible redox reactions .
- Nanomaterials : Research into this compound's interactions with nanomaterials suggests potential applications in sensors and electronic devices, where its conductive properties can be harnessed .
Pharmacological Applications
This compound and its derivatives exhibit significant biological activity, particularly in:
- Antimicrobial Studies : Several studies have indicated that ferrocenyl compounds, including this compound derivatives, possess antimicrobial properties against various pathogens. This has implications for developing new therapeutic agents.
- Anticancer Research : this compound derivatives have shown promise in anticancer studies, with some compounds demonstrating activity against specific cancer cell lines. This aspect is being explored further to understand their mechanisms of action and therapeutic potential.
Electrochemistry
In electrochemical applications, this compound is recognized for its ability to participate in redox reactions. Its applications include:
- Electrochemical Sensors : The compound's redox properties make it suitable for use in electrochemical sensors for detecting various analytes. The incorporation of this compound into sensor designs enhances sensitivity and selectivity .
- Energy Conversion Systems : Research indicates that ferrocene derivatives are leading candidates for applications in energy conversion technologies due to their high thermal stability and favorable electrochemical characteristics .
Case Study 1: Synthesis of Functionalized Ferrocene Derivatives
A study focused on synthesizing various functionalized ferrocene derivatives from this compound demonstrated the versatility of this compound as a building block for complex organic molecules. The research revealed that by modifying the substituents on the cyclopentadienyl ring, different catalytic properties could be achieved, making these derivatives suitable for diverse chemical transformations.
Case Study 2: Anticancer Activity
Research conducted on the anticancer activity of this compound derivatives showed promising results against breast cancer cell lines. The study highlighted the mechanism of action involving the induction of apoptosis in cancer cells, paving the way for further exploration of these compounds as potential chemotherapeutic agents.
Mechanism of Action
The mechanism of action of bromoferrocene involves its ability to undergo various chemical transformations, which are facilitated by the presence of the bromine atom and the iron center. The bromine atom can participate in substitution and coupling reactions, while the iron center can undergo oxidation-reduction processes. These reactions enable this compound to interact with various molecular targets and pathways, making it a versatile compound in chemical research .
Comparison with Similar Compounds
Bromoferrocene belongs to the haloferrocene family, which includes chloroferrocene, iodoferrocene, and polyhalogenated derivatives like 1,1′-dithis compound. Below is a detailed comparison based on reactivity, synthetic utility, and electronic properties:
Structural and Physical Properties
Electronic Effects
- Steric and Electronic Tuning: 1,1′-Dithis compound enables the synthesis of tris-phosphine ligands (e.g., bis(2-diphenylphosphinoferrocenyl)phenylphosphine), leveraging its dual substitution sites for steric control .
Reaction Yields and By-Product Profiles
Key Advantages and Limitations
- This compound :
- 1,1′-Dithis compound :
- Iodoferrocene :
Research Findings and Trends
Recent studies highlight this compound’s role in enantioselective catalysis and medicinal chemistry. For example, 1’-bromoferrocene derivatives with dimethylamino groups enable Pd-catalyzed annulations to form planar chiral ferrocenes (28–42% yields, 92–96% ee) . Additionally, trifluoromethylthioferrocene synthesized from this compound shows promise as an antiparasitic agent, though toxicity concerns necessitate alternative synthetic routes .
Biological Activity
Bromoferrocene, a derivative of ferrocene, has garnered attention in medicinal chemistry due to its unique properties and potential biological activities. This article reviews the biological activity of this compound, focusing on its anticancer and antimicrobial properties, synthesis, and mechanisms of action.
Overview of this compound
This compound is characterized by the presence of bromine substituents on the ferrocene structure, which enhances its lipophilicity and facilitates interactions with biological targets. The compound has been explored for various applications, particularly in cancer therapy and as an antimicrobial agent.
Anticancer Activity
Recent studies have highlighted the anticancer potential of this compound and its derivatives. The incorporation of ferrocene into biologically active molecules has been shown to enhance their cytotoxicity against various cancer cell lines.
Case Studies
- Ferrocifen Development : A notable derivative is ferrocifen, synthesized by replacing the phenyl group in tamoxifen with a ferrocene moiety. This compound exhibits significant activity against hormone-dependent cancers, particularly breast cancer cells (MDA-MB-435-S-F) with IC50 values in the low micromolar range .
- Cytotoxicity Assessments : In vitro studies have demonstrated that this compound derivatives exhibit varying degrees of cytotoxicity across different cancer cell lines, including:
Table 1: Cytotoxicity of this compound Derivatives
Compound | Cell Line | IC50 (µM) | Mechanism of Action |
---|---|---|---|
Ferrocifen | MDA-MB-435-S-F | 9.07 | Estrogen receptor modulation |
This compound | SW620 | 15.4 | Induction of oxidative stress |
Ferroquine | HepG2 | 12.5 | Inhibition of mitochondrial function |
Antimicrobial Activity
In addition to anticancer properties, this compound has shown promising antimicrobial activity against various pathogens. Studies indicate that it can disrupt bacterial membranes and inhibit growth.
Research Findings
- Antibacterial Efficacy : this compound has been tested against Gram-positive and Gram-negative bacteria, showing significant inhibition zones in agar diffusion assays.
- Mechanism : The proposed mechanism involves the generation of reactive oxygen species (ROS), leading to oxidative damage in bacterial cells.
Synthesis and Functionalization
The synthesis of this compound has evolved significantly, allowing for the creation of various derivatives with enhanced biological activities. Techniques such as ortho-deprotonation and lithiation have been employed to produce substituted bromoferrocenes efficiently.
Table 2: Synthesis Methods for this compound Derivatives
Method | Yield (%) | Comments |
---|---|---|
Ortho-deprotonation | 75 | Effective for generating multiple substitutions |
Lithiation followed by bromination | 80 | Allows for controlled substitution patterns |
Q & A
Basic Research Questions
Q. What are the optimal synthetic routes for bromoferrocene, and how do reaction conditions influence yield and purity?
this compound is typically synthesized via electrophilic substitution of ferrocene using brominating agents like Br₂ in the presence of Lewis acids (e.g., AlCl₃) . Key factors affecting yield include temperature control (80–100°C), solvent choice (e.g., hexane or dichloromethane), and stoichiometric ratios of reagents. Post-synthesis purification via column chromatography (Al₂O₃ with heptane) is critical to isolate high-purity this compound, as residual halides or unreacted ferrocene can complicate downstream applications .
Q. How can spectroscopic techniques (NMR, IR) be utilized to confirm this compound’s structure and monitor reaction progress?
- ¹H NMR : this compound’s cyclopentadienyl (Cp) protons exhibit distinct splitting patterns. The monosubstituted derivative shows two doublets (δ 4.2–4.8 ppm), while disubstituted derivatives display singlet peaks due to symmetry.
- IR Spectroscopy : C-Br stretching vibrations appear at ~550–600 cm⁻¹. Comparative analysis with ferrocene’s IR spectrum (absence of Br peaks) confirms successful bromination.
- Mass Spectrometry : Molecular ion peaks at m/z 264.93 (C₁₀H₉BrFe) validate molecular weight .
Q. What are the primary reactivity trends of this compound in cross-coupling or ligand substitution reactions?
this compound undergoes nucleophilic aromatic substitution (e.g., with aryl cuprates) to form aryl-ferrocene derivatives. For example, reacting this compound with Ph₄BCu in pyridine at 125–130°C yields phenyl-ferrocene (56–57% yield). Steric and electronic effects of substituents influence reaction rates and regioselectivity .
Advanced Research Questions
Q. How can computational methods (DFT, molecular modeling) predict this compound’s electronic properties and reactivity in catalysis?
Density Functional Theory (DFT) calculations can model this compound’s frontier molecular orbitals (HOMO/LUMO) to predict redox behavior and ligand-binding affinities. For instance, electron-withdrawing Br substituents lower the HOMO energy, enhancing oxidative stability. These insights guide the design of this compound-based catalysts for asymmetric synthesis or electrochemical applications .
Q. What strategies resolve contradictions in reported catalytic activity data for this compound-derived complexes?
Discrepancies in catalytic performance often arise from variations in ligand coordination modes or solvent effects. Systematic approaches include:
- Controlled Replication : Reproduce studies under identical conditions (solvent, temperature, catalyst loading).
- Operando Spectroscopy : Monitor catalytic intermediates in real-time using techniques like in-situ IR or XAS.
- Meta-Analysis : Compare datasets across literature to identify outliers or methodological biases .
Q. How does steric hindrance from bromine substituents influence this compound’s coordination chemistry in multinuclear complexes?
Bromine’s bulkiness can restrict ligand binding to metal centers. For example, in phosphine-substituted bromoferrocenes (e.g., 1,1’-bis(diisopropylphosphino)this compound), steric clashes between Br and P(iPr)₂ groups reduce flexibility, favoring rigid geometries. X-ray crystallography and variable-temperature NMR are critical to map conformational dynamics .
Q. Methodological and Safety Considerations
Q. What safety protocols are essential for handling this compound in laboratory settings?
this compound is toxic and air-sensitive. Key precautions include:
- Use gloveboxes or Schlenk lines for synthesis/purification.
- Avoid inhalation/contact; wear nitrile gloves and lab coats.
- Store under inert gas (Ar/N₂) at –20°C to prevent decomposition .
Q. How can researchers design a scoping review to identify gaps in this compound’s applications?
Follow Arksey & O’Malley’s framework :
- Step 1 : Define objectives (e.g., “Map this compound’s role in organometallic catalysis”).
- Step 2 : Search databases (SciFinder, Reaxys) using keywords: “bromo-ferrocene,” “cross-coupling,” “ligand design.”
- Step 3 : Chart data (e.g., reaction types, yields, limitations).
- Step 4 : Consult experts to validate findings and prioritize future studies.
Q. Data Presentation Guidelines
Properties
CAS No. |
1273-73-0 |
---|---|
Molecular Formula |
C10H9BrFe 10* |
Molecular Weight |
264.93 |
Origin of Product |
United States |
Disclaimer and Information on In-Vitro Research Products
Please be aware that all articles and product information presented on BenchChem are intended solely for informational purposes. The products available for purchase on BenchChem are specifically designed for in-vitro studies, which are conducted outside of living organisms. In-vitro studies, derived from the Latin term "in glass," involve experiments performed in controlled laboratory settings using cells or tissues. It is important to note that these products are not categorized as medicines or drugs, and they have not received approval from the FDA for the prevention, treatment, or cure of any medical condition, ailment, or disease. We must emphasize that any form of bodily introduction of these products into humans or animals is strictly prohibited by law. It is essential to adhere to these guidelines to ensure compliance with legal and ethical standards in research and experimentation.