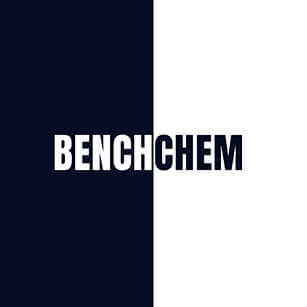
1,1/'-FERROCENEDICARBOXALDEHYDE
- Click on QUICK INQUIRY to receive a quote from our team of experts.
- With the quality product at a COMPETITIVE price, you can focus more on your research.
Overview
Description
Mechanism of Action
Target of Action
1,1’-Ferrocenedicarboxaldehyde, also known as 1,1’-Bisformylferrocene or 1,1’-Diformylferrocene , is a compound that has been used in various applications.
Mode of Action
It’s known that the compound can be used as monomers to synthesize covalent organic framework (cof) materials .
Biochemical Pathways
Its use in the synthesis of cof materials suggests it may play a role in the formation of these complex structures .
Result of Action
Its use in the synthesis of cof materials suggests it may contribute to the formation of these complex structures .
Action Environment
It’s known that the compound should be stored at 2-8℃ , suggesting that temperature may play a role in its stability.
Preparation Methods
1,1/'-Ferrocenedicarboxaldehyde can be synthesized through several methods. One common synthetic route involves the reaction of ferrocene with formylating agents such as N-formylpiperidine . The reaction typically occurs under inert conditions using solvents like hexane and reagents such as n-butyllithium and tetramethylethylenediamine (TMEDA) . The reaction conditions are carefully controlled to ensure the selective formation of the desired product.
Industrial production methods for this compound are similar to laboratory-scale syntheses but are optimized for larger-scale operations. These methods often involve continuous flow reactors and automated systems to maintain consistent reaction conditions and high yields .
Chemical Reactions Analysis
1,1/'-Ferrocenedicarboxaldehyde undergoes various chemical reactions, including oxidation, reduction, and substitution reactions.
The major products formed from these reactions depend on the specific reagents and conditions used. For example, oxidation with potassium permanganate yields carboxylic acids, while reduction with sodium borohydride produces alcohols .
Scientific Research Applications
1,1/'-Ferrocenedicarboxaldehyde has a wide range of applications in scientific research:
Comparison with Similar Compounds
1,1/'-Ferrocenedicarboxaldehyde can be compared with other ferrocene derivatives such as:
Ferrocenecarboxaldehyde (C11H10FeO): This compound has a single formyl group and is used in similar applications but offers different reactivity due to the presence of only one formyl group.
Ferrocenemethanol (C11H12FeO): This derivative has a hydroxymethyl group instead of a formyl group, leading to different chemical properties and applications.
1,1’-Diacetylferrocene (C14H14FeO2):
The uniqueness of this compound lies in its two formyl groups, which provide multiple sites for chemical modification and make it a versatile building block in synthetic chemistry .
Properties
CAS No. |
1271-48-3 |
---|---|
Molecular Formula |
C12H10FeO2 10* |
Molecular Weight |
242.05 |
Origin of Product |
United States |
Disclaimer and Information on In-Vitro Research Products
Please be aware that all articles and product information presented on BenchChem are intended solely for informational purposes. The products available for purchase on BenchChem are specifically designed for in-vitro studies, which are conducted outside of living organisms. In-vitro studies, derived from the Latin term "in glass," involve experiments performed in controlled laboratory settings using cells or tissues. It is important to note that these products are not categorized as medicines or drugs, and they have not received approval from the FDA for the prevention, treatment, or cure of any medical condition, ailment, or disease. We must emphasize that any form of bodily introduction of these products into humans or animals is strictly prohibited by law. It is essential to adhere to these guidelines to ensure compliance with legal and ethical standards in research and experimentation.