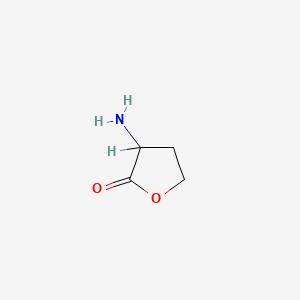
Homoserine lactone
Overview
Description
Homoserine lactone is a chemical compound that plays a significant role in bacterial communication, particularly in quorum sensing. Quorum sensing is a mechanism by which bacteria regulate gene expression in response to changes in cell population density. This compound is an intermediate in the biosynthesis of essential amino acids such as methionine, threonine, and isoleucine .
Preparation Methods
Synthetic Routes and Reaction Conditions: Homoserine lactone can be synthesized from methionine. Methionine reacts with bromoacetic acid in a 20% acetic acid solution of water and isopropanol at reflux temperature. The resultant homoserine hydrobromide is then cyclized by treatment with 4 M hydrochloric acid in dioxane .
Industrial Production Methods: Industrial production methods for this compound typically involve the use of bacterial cultures that naturally produce this compound. The bacteria are cultured under controlled conditions, and the this compound is extracted and purified using various chromatographic techniques .
Chemical Reactions Analysis
Types of Reactions: Homoserine lactone undergoes several types of chemical reactions, including:
Oxidation: this compound can be oxidized to form various oxidized derivatives.
Reduction: It can be reduced to form reduced derivatives.
Substitution: this compound can undergo substitution reactions where different functional groups replace the lactone ring.
Common Reagents and Conditions:
Oxidation: Common oxidizing agents include potassium permanganate and hydrogen peroxide.
Reduction: Common reducing agents include sodium borohydride and lithium aluminum hydride.
Substitution: Common reagents for substitution reactions include halogens and nucleophiles.
Major Products: The major products formed from these reactions depend on the specific reagents and conditions used. For example, oxidation can produce various oxidized forms of this compound, while reduction can yield reduced forms .
Scientific Research Applications
Homoserine lactone has numerous scientific research applications, including:
Chemistry: It is used as a model compound in studying chemical reactions and mechanisms.
Biology: this compound is crucial in studying bacterial communication and quorum sensing.
Industry: this compound is used in the production of biofilms and other bacterial products
Mechanism of Action
Homoserine lactone exerts its effects through quorum sensing. It acts as an autoinducer, a signaling molecule that bacteria produce and release into their environment. When the concentration of this compound reaches a certain threshold, it binds to specific receptor proteins, such as LuxR-type proteins, in the bacterial cells. This binding activates the transcription of target genes, leading to changes in bacterial behavior, such as biofilm formation, virulence, and bioluminescence .
Comparison with Similar Compounds
Homoserine lactone is part of a broader class of compounds known as N-acyl homoserine lactones. These compounds share a similar structure but differ in the length and saturation of their acyl side chains. Similar compounds include:
- N-3-oxo-hexanoyl-homoserine lactone
- N-3-oxododecanoyl-homoserine lactone
- N-butyryl-homoserine lactone
This compound is unique in its specific role in quorum sensing and its ability to regulate a wide range of bacterial behaviors .
Properties
IUPAC Name |
3-aminooxolan-2-one | |
---|---|---|
Source | PubChem | |
URL | https://pubchem.ncbi.nlm.nih.gov | |
Description | Data deposited in or computed by PubChem | |
InChI |
InChI=1S/C4H7NO2/c5-3-1-2-7-4(3)6/h3H,1-2,5H2 | |
Source | PubChem | |
URL | https://pubchem.ncbi.nlm.nih.gov | |
Description | Data deposited in or computed by PubChem | |
InChI Key |
QJPWUUJVYOJNMH-UHFFFAOYSA-N | |
Source | PubChem | |
URL | https://pubchem.ncbi.nlm.nih.gov | |
Description | Data deposited in or computed by PubChem | |
Canonical SMILES |
C1COC(=O)C1N | |
Source | PubChem | |
URL | https://pubchem.ncbi.nlm.nih.gov | |
Description | Data deposited in or computed by PubChem | |
Molecular Formula |
C4H7NO2 | |
Source | PubChem | |
URL | https://pubchem.ncbi.nlm.nih.gov | |
Description | Data deposited in or computed by PubChem | |
Related CAS |
6305-38-0 (hydrobromide) | |
Record name | Homoserine lactone | |
Source | ChemIDplus | |
URL | https://pubchem.ncbi.nlm.nih.gov/substance/?source=chemidplus&sourceid=0001192207 | |
Description | ChemIDplus is a free, web search system that provides access to the structure and nomenclature authority files used for the identification of chemical substances cited in National Library of Medicine (NLM) databases, including the TOXNET system. | |
DSSTOX Substance ID |
DTXSID00862589 | |
Record name | 3-Aminooxolan-2-one | |
Source | EPA DSSTox | |
URL | https://comptox.epa.gov/dashboard/DTXSID00862589 | |
Description | DSSTox provides a high quality public chemistry resource for supporting improved predictive toxicology. | |
Molecular Weight |
101.10 g/mol | |
Source | PubChem | |
URL | https://pubchem.ncbi.nlm.nih.gov | |
Description | Data deposited in or computed by PubChem | |
CAS No. |
1192-20-7 | |
Record name | Homoserine lactone | |
Source | CAS Common Chemistry | |
URL | https://commonchemistry.cas.org/detail?cas_rn=1192-20-7 | |
Description | CAS Common Chemistry is an open community resource for accessing chemical information. Nearly 500,000 chemical substances from CAS REGISTRY cover areas of community interest, including common and frequently regulated chemicals, and those relevant to high school and undergraduate chemistry classes. This chemical information, curated by our expert scientists, is provided in alignment with our mission as a division of the American Chemical Society. | |
Explanation | The data from CAS Common Chemistry is provided under a CC-BY-NC 4.0 license, unless otherwise stated. | |
Record name | Homoserine lactone | |
Source | ChemIDplus | |
URL | https://pubchem.ncbi.nlm.nih.gov/substance/?source=chemidplus&sourceid=0001192207 | |
Description | ChemIDplus is a free, web search system that provides access to the structure and nomenclature authority files used for the identification of chemical substances cited in National Library of Medicine (NLM) databases, including the TOXNET system. | |
Record name | 3-Aminooxolan-2-one | |
Source | EPA DSSTox | |
URL | https://comptox.epa.gov/dashboard/DTXSID00862589 | |
Description | DSSTox provides a high quality public chemistry resource for supporting improved predictive toxicology. | |
Disclaimer and Information on In-Vitro Research Products
Please be aware that all articles and product information presented on BenchChem are intended solely for informational purposes. The products available for purchase on BenchChem are specifically designed for in-vitro studies, which are conducted outside of living organisms. In-vitro studies, derived from the Latin term "in glass," involve experiments performed in controlled laboratory settings using cells or tissues. It is important to note that these products are not categorized as medicines or drugs, and they have not received approval from the FDA for the prevention, treatment, or cure of any medical condition, ailment, or disease. We must emphasize that any form of bodily introduction of these products into humans or animals is strictly prohibited by law. It is essential to adhere to these guidelines to ensure compliance with legal and ethical standards in research and experimentation.