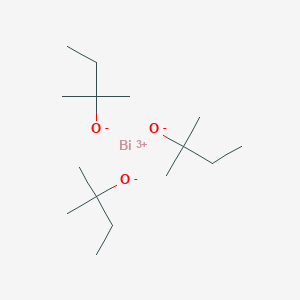
Tris(tert-amyloxy) bismuth
- Click on QUICK INQUIRY to receive a quote from our team of experts.
- With the quality product at a COMPETITIVE price, you can focus more on your research.
Overview
Description
It is a white, odorless, and crystalline solid that is soluble in organic solvents.
Mechanism of Action
Target of Action
Bismuth-based drugs have been primarily used to treat ulcers caused byHelicobacter pylori and other gastrointestinal ailments .
Mode of Action
It’s known that bismuth compounds exhibit antibacterial properties and have a synergistic activity when combined with antibiotics, making them ideal for multiple therapy regimens and overcoming bacterial resistance .
Biochemical Pathways
It’s known that bismuth compounds can inhibit the growth ofH. pylori . The proteome work and in vitro studies all supported that enzyme inhibition, attenuated ROS defense, disruption of the intracellular iron metabolism, and reduced bacterium-host cell adhesion are the principal mechanisms underlying the actions of bismuth against H. pylori .
Result of Action
It’s known that bismuth compounds can be effective against tumoral cells, leishmaniasis, fungi, and viruses .
Biochemical Analysis
Biochemical Properties
Tris(tert-amyloxy) bismuth plays a significant role in biochemical reactions, particularly due to its ability to interact with enzymes, proteins, and other biomolecules. It has been observed to form addition products at the Bi–N bond when reacting with compounds such as 2-benzoylpyridine and 3-pyridinecarboxaldehyde . These interactions suggest that this compound can potentially influence the activity of enzymes and proteins by modifying their structure or function.
Cellular Effects
The effects of this compound on various types of cells and cellular processes are profound. This compound can influence cell function by affecting cell signaling pathways, gene expression, and cellular metabolism. For instance, this compound has been shown to exhibit antibacterial activity, which indicates its potential to disrupt cellular processes in bacterial cells . This disruption can lead to changes in cell signaling and gene expression, ultimately affecting cellular metabolism.
Molecular Mechanism
The molecular mechanism of this compound involves its binding interactions with biomolecules, enzyme inhibition or activation, and changes in gene expression. The compound’s ability to form addition products at the Bi–N bond suggests that it can interact with enzymes and proteins, potentially inhibiting or activating their function . These interactions can lead to changes in gene expression, further influencing cellular processes.
Temporal Effects in Laboratory Settings
In laboratory settings, the effects of this compound can change over time. The stability and degradation of the compound are crucial factors that influence its long-term effects on cellular function. Studies have shown that this compound is stable under certain conditions, but its degradation products can also have significant effects on cells . Long-term exposure to the compound can lead to changes in cellular function, which need to be carefully monitored in in vitro and in vivo studies.
Dosage Effects in Animal Models
The effects of this compound vary with different dosages in animal models. At lower doses, the compound may exhibit beneficial effects, such as antibacterial activity . At higher doses, this compound can have toxic or adverse effects, which need to be carefully evaluated. Threshold effects observed in these studies indicate that there is a fine balance between the beneficial and harmful effects of the compound.
Metabolic Pathways
This compound is involved in various metabolic pathways, interacting with enzymes and cofactors. The compound’s ability to form addition products at the Bi–N bond suggests that it can influence metabolic flux and metabolite levels . These interactions can lead to changes in the activity of enzymes and the levels of metabolites, further affecting cellular metabolism.
Subcellular Localization
The subcellular localization of this compound is crucial for its activity and function. The compound’s ability to form addition products at the Bi–N bond suggests that it can be directed to specific compartments or organelles within the cell . Targeting signals or post-translational modifications may play a role in directing this compound to its site of action, further influencing its biochemical properties.
Preparation Methods
Synthetic Routes and Reaction Conditions: Tris(tert-amyloxy) bismuth is typically synthesized through the reaction of bismuth(III) chloride with tert-amyloxy lithium or tert-amyloxy magnesium reagents. The reaction is carried out in an inert atmosphere, often using solvents like tetrahydrofuran (THF) to facilitate the reaction . The general reaction scheme is as follows:
BiCl3+3(tert−amyloxy)Li→Bi(O−tert−amyloxy)3+3LiCl
The reaction conditions usually involve low temperatures to prevent decomposition and to ensure high yields .
Industrial Production Methods: Industrial production of this compound follows similar synthetic routes but on a larger scale. The process involves the use of large reactors and precise control of reaction conditions to maintain product quality and yield. The use of automated systems for reagent addition and temperature control is common in industrial settings .
Chemical Reactions Analysis
Types of Reactions: Tris(tert-amyloxy) bismuth undergoes various types of chemical reactions, including:
Oxidation: The compound can be oxidized to form bismuth oxides.
Reduction: It can be reduced to elemental bismuth under specific conditions.
Substitution: The tert-amyloxy groups can be substituted with other ligands, such as halides or other alkoxides.
Common Reagents and Conditions:
Oxidation: Common oxidizing agents include oxygen and hydrogen peroxide.
Reduction: Reducing agents like hydrogen gas or hydrazine are used.
Substitution: Halides like chlorine or bromine can be used for substitution reactions.
Major Products Formed:
Oxidation: Bismuth oxides (e.g., Bi2O3).
Reduction: Elemental bismuth.
Substitution: Bismuth halides or other bismuth alkoxides.
Scientific Research Applications
Tris(tert-amyloxy) bismuth has a wide range of applications in scientific research:
Chemistry: It is used as a reagent in organic synthesis and as a catalyst in various chemical reactions.
Biology: The compound has shown potential in biological applications, including as an antimicrobial agent.
Medicine: Bismuth compounds are known for their medicinal properties, and this compound is being explored for its potential in treating gastrointestinal disorders and infections.
Industry: It is used in the production of bismuth-based materials and as a precursor for other bismuth compounds.
Comparison with Similar Compounds
Triphenylbismuth: Another organobismuth compound with similar applications in organic synthesis and catalysis.
Bismuth subsalicylate: Known for its medicinal properties, particularly in treating gastrointestinal disorders.
Bismuth(III) chloride: A common precursor for various bismuth compounds.
Uniqueness: Tris(tert-amyloxy) bismuth is unique due to its specific tert-amyloxy ligands, which provide distinct chemical properties and reactivity compared to other bismuth compounds. Its solubility in organic solvents and stability under various conditions make it particularly valuable in both research and industrial applications.
Properties
IUPAC Name |
bismuth;2-methylbutan-2-olate |
Source
|
---|---|---|
Source | PubChem | |
URL | https://pubchem.ncbi.nlm.nih.gov | |
Description | Data deposited in or computed by PubChem | |
InChI |
InChI=1S/3C5H11O.Bi/c3*1-4-5(2,3)6;/h3*4H2,1-3H3;/q3*-1;+3 |
Source
|
Source | PubChem | |
URL | https://pubchem.ncbi.nlm.nih.gov | |
Description | Data deposited in or computed by PubChem | |
InChI Key |
DRFVTYUXJVLNLR-UHFFFAOYSA-N |
Source
|
Source | PubChem | |
URL | https://pubchem.ncbi.nlm.nih.gov | |
Description | Data deposited in or computed by PubChem | |
Canonical SMILES |
CCC(C)(C)[O-].CCC(C)(C)[O-].CCC(C)(C)[O-].[Bi+3] |
Source
|
Source | PubChem | |
URL | https://pubchem.ncbi.nlm.nih.gov | |
Description | Data deposited in or computed by PubChem | |
Molecular Formula |
C15H33BiO3 |
Source
|
Source | PubChem | |
URL | https://pubchem.ncbi.nlm.nih.gov | |
Description | Data deposited in or computed by PubChem | |
Molecular Weight |
470.40 g/mol |
Source
|
Source | PubChem | |
URL | https://pubchem.ncbi.nlm.nih.gov | |
Description | Data deposited in or computed by PubChem | |
Disclaimer and Information on In-Vitro Research Products
Please be aware that all articles and product information presented on BenchChem are intended solely for informational purposes. The products available for purchase on BenchChem are specifically designed for in-vitro studies, which are conducted outside of living organisms. In-vitro studies, derived from the Latin term "in glass," involve experiments performed in controlled laboratory settings using cells or tissues. It is important to note that these products are not categorized as medicines or drugs, and they have not received approval from the FDA for the prevention, treatment, or cure of any medical condition, ailment, or disease. We must emphasize that any form of bodily introduction of these products into humans or animals is strictly prohibited by law. It is essential to adhere to these guidelines to ensure compliance with legal and ethical standards in research and experimentation.