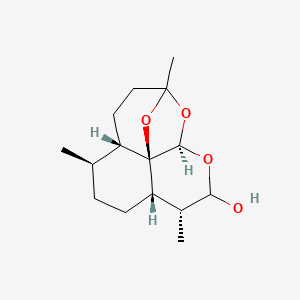
Deoxydihydro-artemisinin
Overview
Description
Deoxydihydro-artemisinin is a derivative of artemisinin, a natural compound extracted from the plant Artemisia annua. Artemisinin and its derivatives, including this compound, are known for their potent antimalarial properties. These compounds have been extensively studied for their efficacy against Plasmodium falciparum, the parasite responsible for the most severe form of malaria .
Preparation Methods
Synthetic Routes and Reaction Conditions: Deoxydihydro-artemisinin is synthesized through the reduction of artemisinin. The process involves the use of reducing agents such as sodium borohydride or lithium aluminum hydride under controlled conditions. The reaction typically takes place in an inert atmosphere to prevent oxidation and ensure high yield .
Industrial Production Methods: Industrial production of this compound follows similar synthetic routes but on a larger scale. The process is optimized for efficiency and cost-effectiveness, often involving continuous flow reactors and advanced purification techniques to achieve high purity and yield .
Chemical Reactions Analysis
Types of Reactions: Deoxydihydro-artemisinin undergoes various chemical reactions, including:
Oxidation: It can be oxidized to form different derivatives, which may have distinct pharmacological properties.
Reduction: Further reduction can lead to the formation of more reduced derivatives.
Substitution: Substitution reactions can introduce different functional groups, altering the compound’s activity and solubility.
Common Reagents and Conditions:
Oxidation: Common oxidizing agents include hydrogen peroxide and potassium permanganate.
Reduction: Sodium borohydride and lithium aluminum hydride are frequently used.
Substitution: Various halogenating agents and nucleophiles are employed depending on the desired substitution.
Major Products Formed: The major products formed from these reactions include various artemisinin derivatives, each with unique pharmacological profiles. These derivatives are often explored for their potential in treating different diseases .
Scientific Research Applications
Deoxydihydro-artemisinin has a wide range of scientific research applications:
Chemistry: It serves as a precursor for synthesizing other artemisinin derivatives with potential therapeutic uses.
Biology: Studies have shown its effectiveness in inhibiting the growth of various parasites and cancer cells.
Medicine: Beyond its antimalarial properties, this compound is being investigated for its anticancer, antiviral, and anti-inflammatory activities.
Industry: It is used in the development of new pharmaceuticals and as a model compound for studying the pharmacokinetics and pharmacodynamics of artemisinin derivatives .
Mechanism of Action
The mechanism of action of deoxydihydro-artemisinin involves the generation of reactive oxygen species (ROS) through the cleavage of its endoperoxide bridge. This leads to the formation of free radicals that damage cellular components of the target organism. The compound targets multiple molecular pathways, including apoptosis-related proteins (BAX, FASL, caspase-3), multi-drug resistance genes, and inflammation-related molecules (NF-κB, COX2) .
Comparison with Similar Compounds
Dihydroartemisinin: Another derivative of artemisinin with similar antimalarial properties.
Artemether: A methyl ether derivative of dihydroartemisinin, used in combination therapies for malaria.
Artesunate: A water-soluble derivative used for severe malaria treatment.
Artemisinin B and G: Other derivatives with varying degrees of efficacy and toxicity
Uniqueness: Deoxydihydro-artemisinin stands out due to its unique balance of efficacy and safety. It has shown lower toxicity compared to some other derivatives while maintaining potent antimalarial and anticancer activities. This makes it a valuable compound for further research and therapeutic development .
Biological Activity
Deoxydihydro-artemisinin (DHA) is a semi-synthetic derivative of artemisinin, a compound derived from the plant Artemisia annua, widely recognized for its antimalarial properties. This article delves into the biological activity of this compound, focusing on its mechanisms of action, therapeutic applications, and relevant research findings.
The biological activity of this compound primarily involves its interaction with the malaria parasite Plasmodium falciparum. It operates through several mechanisms:
- Protein Damage : this compound induces oxidative stress within the parasite, leading to protein damage. This occurs via the generation of reactive oxygen species (ROS) that disrupt protein synthesis and function, ultimately causing cell death .
- Alkylation of Heme : The compound is believed to alkylate heme, a process that releases free radicals. These radicals further contribute to cellular damage within the parasite, enhancing the drug's efficacy .
- Inhibition of Proteasome Function : this compound compromises the proteasome function in Plasmodium by accumulating polyubiquitinated proteins, which triggers an endoplasmic reticulum (ER) stress response that is lethal to the parasite .
Antimalarial Activity
Research has demonstrated that this compound exhibits potent antimalarial activity. In vitro studies have shown that it has a half-maximal inhibitory concentration (IC50) ranging from 6 to 223 nM against various strains of P. falciparum. This indicates a strong capacity to inhibit the growth of malaria parasites.
Comparative Antimalarial Efficacy
Compound | IC50 (nM) |
---|---|
This compound | 6 - 223 |
Dihydroartemisinin | 10 - 50 |
Artemisinin | 5 - 30 |
Case Studies and Research Findings
- Biotransformation Studies : A study involving biotransformation of this compound using fungal strains such as Cunninghamella elegans led to the identification of several metabolites with enhanced antimalarial activity. This highlights the potential for developing more effective derivatives through biotechnological methods .
- Toxicity Assessment : Recent machine learning-based assessments have indicated that this compound has a favorable toxicity profile compared to other artemisinin derivatives. In silico predictions showed low cardiotoxicity and minimal hepatotoxicity, making it a safer alternative for therapeutic use .
- In Vivo Efficacy : Animal studies have confirmed that this compound maintains high bioavailability and efficacy against malaria in vivo, supporting its potential as a frontline treatment option .
Properties
IUPAC Name |
(4S,5R,8S,9R,12R,13R)-1,5,9-trimethyl-11,14,15-trioxatetracyclo[10.2.1.04,13.08,13]pentadecan-10-ol | |
---|---|---|
Source | PubChem | |
URL | https://pubchem.ncbi.nlm.nih.gov | |
Description | Data deposited in or computed by PubChem | |
InChI |
InChI=1S/C15H24O4/c1-8-4-5-11-9(2)12(16)17-13-15(11)10(8)6-7-14(3,18-13)19-15/h8-13,16H,4-7H2,1-3H3/t8-,9-,10+,11+,12?,13-,14?,15-/m1/s1 | |
Source | PubChem | |
URL | https://pubchem.ncbi.nlm.nih.gov | |
Description | Data deposited in or computed by PubChem | |
InChI Key |
JQGOBHOUYKYFPD-YONALABKSA-N | |
Source | PubChem | |
URL | https://pubchem.ncbi.nlm.nih.gov | |
Description | Data deposited in or computed by PubChem | |
Canonical SMILES |
CC1CCC2C(C(OC3C24C1CCC(O3)(O4)C)O)C | |
Source | PubChem | |
URL | https://pubchem.ncbi.nlm.nih.gov | |
Description | Data deposited in or computed by PubChem | |
Isomeric SMILES |
C[C@@H]1CC[C@H]2[C@H](C(O[C@H]3[C@@]24[C@H]1CCC(O3)(O4)C)O)C | |
Source | PubChem | |
URL | https://pubchem.ncbi.nlm.nih.gov | |
Description | Data deposited in or computed by PubChem | |
Molecular Formula |
C15H24O4 | |
Source | PubChem | |
URL | https://pubchem.ncbi.nlm.nih.gov | |
Description | Data deposited in or computed by PubChem | |
Molecular Weight |
268.35 g/mol | |
Source | PubChem | |
URL | https://pubchem.ncbi.nlm.nih.gov | |
Description | Data deposited in or computed by PubChem | |
CAS No. |
72807-92-2 | |
Record name | Dihydrodeoxyartemisinin | |
Source | ChemIDplus | |
URL | https://pubchem.ncbi.nlm.nih.gov/substance/?source=chemidplus&sourceid=0072807922 | |
Description | ChemIDplus is a free, web search system that provides access to the structure and nomenclature authority files used for the identification of chemical substances cited in National Library of Medicine (NLM) databases, including the TOXNET system. | |
Record name | DIHYDRODEOXYARTEMISININ | |
Source | FDA Global Substance Registration System (GSRS) | |
URL | https://gsrs.ncats.nih.gov/ginas/app/beta/substances/MF8A49NO18 | |
Description | The FDA Global Substance Registration System (GSRS) enables the efficient and accurate exchange of information on what substances are in regulated products. Instead of relying on names, which vary across regulatory domains, countries, and regions, the GSRS knowledge base makes it possible for substances to be defined by standardized, scientific descriptions. | |
Explanation | Unless otherwise noted, the contents of the FDA website (www.fda.gov), both text and graphics, are not copyrighted. They are in the public domain and may be republished, reprinted and otherwise used freely by anyone without the need to obtain permission from FDA. Credit to the U.S. Food and Drug Administration as the source is appreciated but not required. | |
Q1: What is the significance of the endoperoxide bridge in artemisinin derivatives for their cytotoxic activity against tumor cells?
A1: While the endoperoxide bridge is often considered crucial for the antimalarial activity of artemisinin and its derivatives, research suggests it might not be essential for cytotoxicity against tumor cells. A study comparing the cytotoxicity of various artemisinin derivatives against EN2 tumor cells found that while artemisinin (containing the endoperoxide bridge) was more cytotoxic than deoxyartemisinin (lacking the bridge), both nonsymmetrical and symmetrical dimers of dihydrodeoxyartemisinin (lacking the bridge) still exhibited cytotoxic effects. [] This suggests that other structural features, such as the ether linkage in dimers, might play a significant role in mediating cytotoxicity against tumor cells.
Q2: How does the stereochemistry of dihydrodeoxyartemisinin dimers affect their cytotoxic activity?
A2: Research indicates that the stereochemistry of dihydrodeoxyartemisinin dimers significantly influences their cytotoxic activity. In a study using EN2 tumor cells, the nonsymmetrical dimer of dihydrodeoxyartemisinin demonstrated higher cytotoxicity compared to its symmetrical counterpart. [] This difference in activity highlights the importance of spatial arrangement and its impact on the interaction of these compounds with their biological targets.
Q3: What is the impact of dihydroartemisinin dimers on the cell cycle of tumor cells?
A3: Studies show that dihydroartemisinin dimers can disrupt the cell cycle progression of tumor cells. Flow cytometry analysis revealed that both symmetrical and nonsymmetrical dihydroartemisinin dimers caused an accumulation of EN2 cells in the G1 phase of the cell cycle. [] This interruption of the cell cycle suggests a potential mechanism by which these compounds exert their cytotoxic effects, potentially pushing the cells towards programmed cell death or inhibiting their proliferation.
Disclaimer and Information on In-Vitro Research Products
Please be aware that all articles and product information presented on BenchChem are intended solely for informational purposes. The products available for purchase on BenchChem are specifically designed for in-vitro studies, which are conducted outside of living organisms. In-vitro studies, derived from the Latin term "in glass," involve experiments performed in controlled laboratory settings using cells or tissues. It is important to note that these products are not categorized as medicines or drugs, and they have not received approval from the FDA for the prevention, treatment, or cure of any medical condition, ailment, or disease. We must emphasize that any form of bodily introduction of these products into humans or animals is strictly prohibited by law. It is essential to adhere to these guidelines to ensure compliance with legal and ethical standards in research and experimentation.