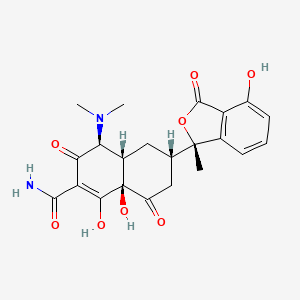
Isotetracycline
- Click on QUICK INQUIRY to receive a quote from our team of experts.
- With the quality product at a COMPETITIVE price, you can focus more on your research.
Overview
Description
Isotetracycline is a derivative of tetracycline, a broad-spectrum antibiotic that belongs to the class of polyketides. It is known for its ability to inhibit bacterial protein synthesis, making it effective against a wide range of bacterial infections. This compound is particularly notable for its structural modifications, which enhance its stability and efficacy compared to its parent compound, tetracycline .
Mechanism of Action
Target of Action
Isotetracycline, like other tetracycline antibiotics, primarily targets bacterial cells . The primary targets are the 30S ribosomal subunits of the bacteria . These subunits play a crucial role in protein synthesis, which is vital for bacterial growth and survival .
Mode of Action
This compound exerts its antibacterial effect by binding reversibly to the bacterial 30S ribosomal subunit . This binding prevents the incoming aminoacyl-tRNA from attaching to the ribosome acceptor site, thereby interfering with protein synthesis . It also binds to some extent to the bacterial 50S ribosomal subunit and may alter the cytoplasmic membrane, causing intracellular components to leak from bacterial cells .
Biochemical Pathways
The primary biochemical pathway affected by this compound is the protein synthesis pathway in bacteria . By inhibiting this pathway, this compound prevents the bacteria from producing essential proteins, leading to a halt in bacterial growth . The downstream effects include disruption of various cellular functions and eventual death of the bacteria .
Pharmacokinetics
Tetracyclines, in general, are known for their broad-spectrum activity and are absorbed well in the gastrointestinal tract . They are distributed widely in body tissues and fluids, metabolized in the liver, and excreted primarily in urine . These properties impact the bioavailability of this compound, determining its effectiveness in treating infections.
Result of Action
The molecular effect of this compound involves the disruption of protein synthesis, leading to a halt in bacterial growth . On a cellular level, this can cause changes in the bacterial cell membrane and leakage of intracellular components . The overall result is a bacteriostatic effect, where the growth of bacteria is inhibited, allowing the immune system to eliminate the infection .
Action Environment
Environmental factors can significantly influence the action, efficacy, and stability of this compound. For instance, the presence of this compound in the environment can lead to the development of antibiotic-resistant bacteria . Moreover, ineffective wastewater treatment systems can lead to antibiotic contamination of the food chain, potentially affecting human health . Therefore, proper management and disposal of this compound are crucial to minimize its environmental impact and maintain its efficacy.
Biochemical Analysis
Biochemical Properties
Isotetracycline interacts with several biomolecules in its biochemical reactions. It forms to anhydrotetracyclines at low pH, 4-epitetracyclines at pH 3–5, and isotetracyclines at high pH values . The transformation of this compound at basic pH is determined by absorption, emission, and time-resolved spectroscopy .
Cellular Effects
This compound, like other tetracyclines, has a broad spectrum of activity against various types of cells. It is known to inhibit protein synthesis in both bacterial and human cells . Human cells are spared the effects of this compound on protein synthesis as they do not have a system that allows tetracyclines to be transported into the cell .
Molecular Mechanism
This compound exerts its effects at the molecular level primarily by inhibiting protein synthesis. It binds reversibly to the bacterial 30S ribosomal subunit, preventing the aminoacyl tRNA from binding to the A site of the ribosome . It also binds to some extent to the bacterial 50S ribosomal subunit and may alter the cytoplasmic membrane causing intracellular components to leak from bacterial cells .
Temporal Effects in Laboratory Settings
The effects of this compound change over time in laboratory settings. For instance, increasing the buffer’s ionic concentration leads to faster transformation to this compound . A pH-dependent transformation of chlortetracycline to this compound has been observed .
Metabolic Pathways
This compound is involved in several metabolic pathways. It is known to undergo a wide variety of reactions at different pH values
Transport and Distribution
This compound is transported and distributed within cells and tissues. Tetracyclines, including this compound, enter the bacterial cell wall in two ways: passive diffusion and an energy-dependent active transport system, which is probably mediated in a pH-dependent fashion .
Preparation Methods
Synthetic Routes and Reaction Conditions
Isotetracycline can be synthesized through the mild alkali treatment of tetracycline. This process involves the transformation of tetracycline at the 11a carbon position to yield this compound . The reaction typically requires controlled conditions to ensure the selective conversion without degrading the compound.
Industrial Production Methods
On an industrial scale, this compound is produced through a combination of fermentation and chemical synthesis. The initial fermentation process involves the cultivation of Streptomyces bacteria, which produce tetracycline. This is followed by a chemical modification step where tetracycline is treated with mild alkali to produce this compound .
Chemical Reactions Analysis
Types of Reactions
Isotetracycline undergoes several types of chemical reactions, including:
Oxidation: this compound can be oxidized under specific conditions to form various oxidized derivatives.
Reduction: Reduction reactions can modify the functional groups on this compound, altering its chemical properties.
Common Reagents and Conditions
Oxidation: Common oxidizing agents include hydrogen peroxide and potassium permanganate.
Reduction: Reducing agents such as sodium borohydride are often used.
Substitution: Various reagents, including halogens and alkylating agents, are employed in substitution reactions.
Major Products Formed
The major products formed from these reactions include various derivatives of this compound with modified functional groups, which can exhibit different biological activities and pharmacological properties .
Scientific Research Applications
Isotetracycline has a wide range of applications in scientific research, including:
Chemistry: Used as a model compound for studying the synthesis and modification of polyketides.
Biology: Employed in research on bacterial resistance mechanisms and protein synthesis inhibition.
Medicine: Investigated for its potential use in treating bacterial infections resistant to other antibiotics.
Industry: Utilized in the development of new antibiotics and as a growth promoter in animal feed.
Comparison with Similar Compounds
Similar Compounds
Tetracycline: The parent compound, known for its broad-spectrum antibiotic activity.
Oxytetracycline: Another tetracycline derivative with similar antibacterial properties.
Doxycycline: A more potent and longer-acting tetracycline derivative.
Minocycline: Known for its enhanced lipid solubility and ability to cross the blood-brain barrier.
Uniqueness of Isotetracycline
This compound is unique due to its structural modifications, which confer greater stability and efficacy compared to tetracycline. These modifications also reduce the likelihood of bacterial resistance, making this compound a valuable compound in the fight against antibiotic-resistant infections .
Properties
IUPAC Name |
(4S,4aS,6S,8aR)-4-(dimethylamino)-1,8a-dihydroxy-6-[(1S)-4-hydroxy-1-methyl-3-oxo-2-benzofuran-1-yl]-3,8-dioxo-4a,5,6,7-tetrahydro-4H-naphthalene-2-carboxamide |
Source
|
---|---|---|
Source | PubChem | |
URL | https://pubchem.ncbi.nlm.nih.gov | |
Description | Data deposited in or computed by PubChem | |
InChI |
InChI=1S/C22H24N2O8/c1-21(10-5-4-6-12(25)14(10)20(30)32-21)9-7-11-16(24(2)3)17(27)15(19(23)29)18(28)22(11,31)13(26)8-9/h4-6,9,11,16,25,28,31H,7-8H2,1-3H3,(H2,23,29)/t9-,11-,16-,21-,22-/m0/s1 |
Source
|
Source | PubChem | |
URL | https://pubchem.ncbi.nlm.nih.gov | |
Description | Data deposited in or computed by PubChem | |
InChI Key |
FBFMFLWQGROEOB-GTCCLLRZSA-N |
Source
|
Source | PubChem | |
URL | https://pubchem.ncbi.nlm.nih.gov | |
Description | Data deposited in or computed by PubChem | |
Canonical SMILES |
CC1(C2=C(C(=CC=C2)O)C(=O)O1)C3CC4C(C(=O)C(=C(C4(C(=O)C3)O)O)C(=O)N)N(C)C |
Source
|
Source | PubChem | |
URL | https://pubchem.ncbi.nlm.nih.gov | |
Description | Data deposited in or computed by PubChem | |
Isomeric SMILES |
C[C@@]1(C2=C(C(=CC=C2)O)C(=O)O1)[C@H]3C[C@H]4[C@@H](C(=O)C(=C([C@]4(C(=O)C3)O)O)C(=O)N)N(C)C |
Source
|
Source | PubChem | |
URL | https://pubchem.ncbi.nlm.nih.gov | |
Description | Data deposited in or computed by PubChem | |
Molecular Formula |
C22H24N2O8 |
Source
|
Source | PubChem | |
URL | https://pubchem.ncbi.nlm.nih.gov | |
Description | Data deposited in or computed by PubChem | |
Molecular Weight |
444.4 g/mol |
Source
|
Source | PubChem | |
URL | https://pubchem.ncbi.nlm.nih.gov | |
Description | Data deposited in or computed by PubChem | |
Q1: Can isotetracycline inhibit enzymes like tetracycline antibiotics?
A: While this compound is generally considered inactive as an antibiotic, research shows it can inhibit the metalloflavoenzyme NADH-cytochrome c oxidoreductase []. This inhibition is thought to occur, at least partially, through the chelation of enzyme-bound metal, similar to the proposed mechanism for tetracycline antibiotics []. The potential chelation sites on this compound are believed to be the C-11 to C-12 chromophore or the C-1, C-2, C-3 region involving the 2-carboxamide group [].
Q2: Does the structure of this compound impact its ability to inhibit enzymes compared to tetracyclines?
A: Modifications to the this compound structure, particularly at the 2-carboxamide substituent and the 4-dimethylamino group, have been observed to diminish its inhibitory effect on NADH-cytochrome c oxidoreductase []. This observation mirrors the structure-activity relationships seen with tetracycline antibiotics, suggesting that these specific structural features are crucial for the inhibitory activity of both tetracyclines and this compound [].
Q3: What is the significance of this compound being able to bind to targets and inhibit enzymes despite being a degradation product?
A: The ability of this compound to bind to targets like TetR and inhibit enzymes like NADH-cytochrome c oxidoreductase raises important questions about its potential biological activity [, ]. Although considered inactive as an antibiotic, its interaction with these targets could have unforeseen consequences. Further research is needed to investigate these interactions thoroughly and determine if this compound possesses any significant biological activity or side effects.
Disclaimer and Information on In-Vitro Research Products
Please be aware that all articles and product information presented on BenchChem are intended solely for informational purposes. The products available for purchase on BenchChem are specifically designed for in-vitro studies, which are conducted outside of living organisms. In-vitro studies, derived from the Latin term "in glass," involve experiments performed in controlled laboratory settings using cells or tissues. It is important to note that these products are not categorized as medicines or drugs, and they have not received approval from the FDA for the prevention, treatment, or cure of any medical condition, ailment, or disease. We must emphasize that any form of bodily introduction of these products into humans or animals is strictly prohibited by law. It is essential to adhere to these guidelines to ensure compliance with legal and ethical standards in research and experimentation.