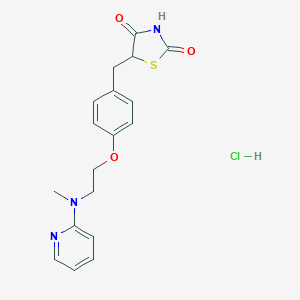
Rosiglitazone hydrochloride
Overview
Description
Rosiglitazone Hydrochloride is a thiazolidinedione class drug primarily used as an anti-diabetic medication. It is marketed under the brand name Avandia and is used to improve glycemic control in adults with type 2 diabetes mellitus. The compound works by increasing the body’s sensitivity to insulin, thereby helping to control blood sugar levels .
Mechanism of Action
Target of Action
Rosiglitazone hydrochloride primarily targets the Peroxisome Proliferator-Activated Receptors (PPARs) , specifically PPARγ . PPARs are intracellular receptors that regulate the transcription of a number of insulin-responsive genes involved in glucose and lipid metabolism . PPARγ is mainly expressed in fat tissue, where it regulates genes involved in fat cell differentiation, fatty acid uptake and storage, and glucose uptake .
Mode of Action
By activating PPARγ, this compound influences the production of gene products involved in glucose and lipid metabolism . This activation improves the target cell’s response to insulin, thereby lowering blood glucose levels without increasing pancreatic insulin secretion . Apart from its effect on insulin resistance, this compound also appears to have an anti-inflammatory effect: nuclear factor kappa-B (NFκB) levels fall and inhibitor (IκB) levels increase in patients on rosiglitazone .
Biochemical Pathways
This compound affects several biochemical pathways. The PI3K/AKT pathway is significantly related to one subnetwork of this compound . Other affected pathways include metabolic pathways, carbon metabolism, and the citrate cycle . These pathways are involved in various cellular processes, including glucose and lipid metabolism, which are crucial for maintaining glycemic control in type 2 diabetes.
Pharmacokinetics
This compound exhibits a high bioavailability of 99% . It is metabolized in the liver, primarily via the CYP2C8 enzyme, with minor metabolism via CYP2C9 . The metabolites are excreted in urine (approximately 64%) and feces (approximately 23%) . The drug has an elimination half-life of 3-4 hours .
Result of Action
The activation of PPARγ by this compound leads to improved insulin sensitivity in target cells, resulting in lower blood glucose levels . This makes this compound effective in maintaining glycemic control in adults with type 2 diabetes . Additionally, it has an anti-inflammatory effect, as evidenced by the decrease in NFκB levels and increase in IκB levels in patients on rosiglitazone .
Action Environment
The action, efficacy, and stability of this compound can be influenced by various environmental factors. This compound is generally associated with bodyweight gain, and it may have detrimental effects on bone
Biochemical Analysis
Biochemical Properties
Rosiglitazone hydrochloride plays a significant role in biochemical reactions by interacting with various enzymes, proteins, and other biomolecules. It is a selective ligand for the peroxisome proliferator-activated receptor gamma (PPARγ), a type of nuclear receptor. By binding to PPARγ, this compound activates the receptor, which in turn regulates the transcription of insulin-responsive genes involved in the control of glucose production, transport, and utilization . This interaction enhances tissue sensitivity to insulin and has anti-inflammatory effects by reducing levels of nuclear factor kappa-B (NFκB) and increasing levels of its inhibitor (IκB) .
Cellular Effects
This compound affects various types of cells and cellular processes. It influences cell function by modulating cell signaling pathways, gene expression, and cellular metabolism. In adipocytes (fat cells), this compound promotes the differentiation of preadipocytes into mature adipocytes, enhancing their ability to store fatty acids and glucose . In muscle and liver cells, it improves insulin sensitivity, leading to increased glucose uptake and decreased glucose production . Additionally, this compound exhibits anti-inflammatory properties by inhibiting the release of pro-inflammatory cytokines such as tumor necrosis factor-alpha (TNFα) and interleukin-6 (IL-6) .
Molecular Mechanism
The molecular mechanism of action of this compound involves its binding to PPARγ receptors. Upon binding, this compound activates PPARγ, which then forms a heterodimer with the retinoid X receptor (RXR). The activation of these genes leads to increased transcription of proteins involved in glucose and lipid metabolism, such as glucose transporter type 4 (GLUT4) and adiponectin . This compound also inhibits the phosphorylation and nuclear translocation of signal transducer and activator of transcription 1 (Stat1) and NFκB, reducing the expression of pro-inflammatory genes .
Temporal Effects in Laboratory Settings
In laboratory settings, the effects of this compound change over time. The stability and degradation of the compound can influence its long-term effects on cellular function. Studies have shown that this compound remains stable under standard laboratory conditions, but its efficacy may decrease over extended periods due to degradation . Long-term exposure to this compound has been associated with sustained improvements in insulin sensitivity and glycemic control in in vitro and in vivo studies . Prolonged use may also lead to adverse effects such as weight gain and fluid retention .
Dosage Effects in Animal Models
The effects of this compound vary with different dosages in animal models. At low to moderate doses, this compound effectively improves insulin sensitivity and glycemic control without significant adverse effects . At high doses, it can cause toxic effects such as hepatotoxicity and cardiovascular complications . Studies in diabetic animal models have shown that this compound reduces fasting glucose levels and glycosylated hemoglobin (HbA1c) levels in a dose-dependent manner .
Metabolic Pathways
This compound is extensively metabolized in the liver by cytochrome P450 enzymes, primarily CYP2C8 and to a lesser extent CYP2C9 . The main metabolic pathways involve N-demethylation and hydroxylation, resulting in the formation of inactive metabolites such as N-desmethyl rosiglitazone and ρ-hydroxy rosiglitazone . These metabolites are further conjugated with sulfate and glucuronic acid before being excreted in the urine and feces .
Transport and Distribution
This compound is transported and distributed within cells and tissues through various mechanisms. It has a high bioavailability and is extensively bound to plasma proteins, primarily albumin . The compound is distributed to target tissues such as adipose tissue, muscle, and liver, where it exerts its effects by activating PPARγ receptors . The distribution of this compound is influenced by its lipophilicity, allowing it to accumulate in lipid-rich tissues .
Subcellular Localization
The subcellular localization of this compound is primarily within the nucleus, where it binds to PPARγ receptors and regulates gene transcription . The compound may also affect the localization of other proteins involved in its signaling pathways. For example, this compound has been shown to influence the subcellular localization of Smad3 and Smad4 in glioma cells, affecting their activity and function . Additionally, post-translational modifications such as phosphorylation can modulate the activity and localization of proteins involved in this compound signaling pathways .
Preparation Methods
The synthesis of Rosiglitazone Hydrochloride involves a five-step synthetic route starting from commercially available materials such as 2-chloropyridine, N-methylethanolamine, 4-fluorobenzaldehyde or 4-hydroxybenzaldehyde, and 1,3-thiazolidine-2,4-dione. The steps include cyclization, alkylation, etherification, condensation, and reduction. The optimal yields for these steps are 90%, 99%, 59%, 75%, and 91%, respectively. The overall yield for the synthesis is approximately 40%, making it suitable for industrial purposes .
Chemical Reactions Analysis
Rosiglitazone Hydrochloride undergoes various chemical reactions, including:
Oxidation: This reaction typically involves the use of oxidizing agents to convert the compound into its oxidized form.
Reduction: Reduction reactions are used to convert the compound into its reduced form, often using reducing agents.
Substitution: This involves replacing one functional group in the compound with another, using specific reagents and conditions. Common reagents used in these reactions include oxidizing agents like potassium permanganate, reducing agents like sodium borohydride, and various catalysts for substitution reactions.
Scientific Research Applications
Rosiglitazone Hydrochloride has a wide range of scientific research applications:
Chemistry: It is used in the study of chemical reactions and synthesis methods.
Biology: The compound is used to study cellular mechanisms and pathways, particularly those related to insulin sensitivity and glucose metabolism.
Medicine: this compound is extensively used in the treatment of type 2 diabetes mellitus. .
Industry: The compound is used in the pharmaceutical industry for the production of anti-diabetic medications.
Comparison with Similar Compounds
Rosiglitazone Hydrochloride is often compared with other thiazolidinediones, such as Pioglitazone. Both compounds work by activating PPARγ and improving insulin sensitivity. this compound has been associated with a higher risk of cardiovascular events compared to Pioglitazone. Despite this, it remains a valuable medication for managing type 2 diabetes mellitus .
Similar Compounds
- Pioglitazone
- Troglitazone
- Ciglitazone
These compounds share similar mechanisms of action but differ in their pharmacokinetic profiles and side effect profiles .
Properties
IUPAC Name |
5-[[4-[2-[methyl(pyridin-2-yl)amino]ethoxy]phenyl]methyl]-1,3-thiazolidine-2,4-dione;hydrochloride | |
---|---|---|
Source | PubChem | |
URL | https://pubchem.ncbi.nlm.nih.gov | |
Description | Data deposited in or computed by PubChem | |
InChI |
InChI=1S/C18H19N3O3S.ClH/c1-21(16-4-2-3-9-19-16)10-11-24-14-7-5-13(6-8-14)12-15-17(22)20-18(23)25-15;/h2-9,15H,10-12H2,1H3,(H,20,22,23);1H | |
Source | PubChem | |
URL | https://pubchem.ncbi.nlm.nih.gov | |
Description | Data deposited in or computed by PubChem | |
InChI Key |
XRSCTTPDKURIIJ-UHFFFAOYSA-N | |
Source | PubChem | |
URL | https://pubchem.ncbi.nlm.nih.gov | |
Description | Data deposited in or computed by PubChem | |
Canonical SMILES |
CN(CCOC1=CC=C(C=C1)CC2C(=O)NC(=O)S2)C3=CC=CC=N3.Cl | |
Source | PubChem | |
URL | https://pubchem.ncbi.nlm.nih.gov | |
Description | Data deposited in or computed by PubChem | |
Molecular Formula |
C18H20ClN3O3S | |
Source | PubChem | |
URL | https://pubchem.ncbi.nlm.nih.gov | |
Description | Data deposited in or computed by PubChem | |
DSSTOX Substance ID |
DTXSID90432078 | |
Record name | Rosiglitazone hydrochloride | |
Source | EPA DSSTox | |
URL | https://comptox.epa.gov/dashboard/DTXSID90432078 | |
Description | DSSTox provides a high quality public chemistry resource for supporting improved predictive toxicology. | |
Molecular Weight |
393.9 g/mol | |
Source | PubChem | |
URL | https://pubchem.ncbi.nlm.nih.gov | |
Description | Data deposited in or computed by PubChem | |
CAS No. |
302543-62-0 | |
Record name | Rosiglitazone hydrochloride | |
Source | ChemIDplus | |
URL | https://pubchem.ncbi.nlm.nih.gov/substance/?source=chemidplus&sourceid=0302543620 | |
Description | ChemIDplus is a free, web search system that provides access to the structure and nomenclature authority files used for the identification of chemical substances cited in National Library of Medicine (NLM) databases, including the TOXNET system. | |
Record name | Rosiglitazone hydrochloride | |
Source | EPA DSSTox | |
URL | https://comptox.epa.gov/dashboard/DTXSID90432078 | |
Description | DSSTox provides a high quality public chemistry resource for supporting improved predictive toxicology. | |
Record name | ROSIGLITAZONE HYDROCHLORIDE | |
Source | FDA Global Substance Registration System (GSRS) | |
URL | https://gsrs.ncats.nih.gov/ginas/app/beta/substances/S3055SS582 | |
Description | The FDA Global Substance Registration System (GSRS) enables the efficient and accurate exchange of information on what substances are in regulated products. Instead of relying on names, which vary across regulatory domains, countries, and regions, the GSRS knowledge base makes it possible for substances to be defined by standardized, scientific descriptions. | |
Explanation | Unless otherwise noted, the contents of the FDA website (www.fda.gov), both text and graphics, are not copyrighted. They are in the public domain and may be republished, reprinted and otherwise used freely by anyone without the need to obtain permission from FDA. Credit to the U.S. Food and Drug Administration as the source is appreciated but not required. | |
Synthesis routes and methods
Procedure details
Retrosynthesis Analysis
AI-Powered Synthesis Planning: Our tool employs the Template_relevance Pistachio, Template_relevance Bkms_metabolic, Template_relevance Pistachio_ringbreaker, Template_relevance Reaxys, Template_relevance Reaxys_biocatalysis model, leveraging a vast database of chemical reactions to predict feasible synthetic routes.
One-Step Synthesis Focus: Specifically designed for one-step synthesis, it provides concise and direct routes for your target compounds, streamlining the synthesis process.
Accurate Predictions: Utilizing the extensive PISTACHIO, BKMS_METABOLIC, PISTACHIO_RINGBREAKER, REAXYS, REAXYS_BIOCATALYSIS database, our tool offers high-accuracy predictions, reflecting the latest in chemical research and data.
Strategy Settings
Precursor scoring | Relevance Heuristic |
---|---|
Min. plausibility | 0.01 |
Model | Template_relevance |
Template Set | Pistachio/Bkms_metabolic/Pistachio_ringbreaker/Reaxys/Reaxys_biocatalysis |
Top-N result to add to graph | 6 |
Feasible Synthetic Routes
Disclaimer and Information on In-Vitro Research Products
Please be aware that all articles and product information presented on BenchChem are intended solely for informational purposes. The products available for purchase on BenchChem are specifically designed for in-vitro studies, which are conducted outside of living organisms. In-vitro studies, derived from the Latin term "in glass," involve experiments performed in controlled laboratory settings using cells or tissues. It is important to note that these products are not categorized as medicines or drugs, and they have not received approval from the FDA for the prevention, treatment, or cure of any medical condition, ailment, or disease. We must emphasize that any form of bodily introduction of these products into humans or animals is strictly prohibited by law. It is essential to adhere to these guidelines to ensure compliance with legal and ethical standards in research and experimentation.