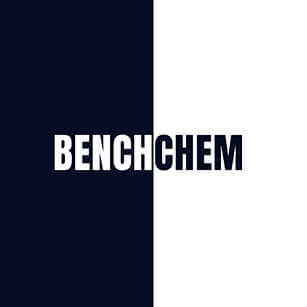
TITANIUM OXYSULFATE
- Click on QUICK INQUIRY to receive a quote from our team of experts.
- With the quality product at a COMPETITIVE price, you can focus more on your research.
Overview
Description
Titanium oxysulfate, also known as titanium(IV) oxysulfate, is a chemical compound with the formula TiOSO₄. It is a white or slightly yellow powder that is highly soluble in water and exhibits hygroscopic properties. This compound is commonly used as a precursor in the production of titanium dioxide, which is widely utilized in various industrial applications such as pigments, photocatalysts, and coatings.
Mechanism of Action
Target of Action
Titanium Oxysulfate (TiOSO4) primarily targets the process of synthesizing Titanium Dioxide (TiO2) from ilmenite . TiO2 is a compound with various strategic applications, including dye-sensitized solar cells, photocatalysts, and water splitting .
Mode of Action
The mode of action of this compound involves its role as a precursor in the synthesis of TiO2 . The process entails the dissolution of ilmenite ore (FeTiO3) in aqueous sulfuric acid, which precipitates the Fe while retaining the Ti in solution . This is followed by dilution or heating of the Ti sulfate solution to precipitate the pure form of TiO2 .
Biochemical Pathways
The biochemical pathway involved in the action of this compound is the conversion of ilmenite into TiO2 . This process involves a hydrothermal reaction, water leaching at 40 °C, and sulfuric acid leaching with two different concentrations of 3 and 18 M at 90 °C . The effectiveness of these treatments is noticeable as the grain size becomes smaller after water and acid leaching .
Result of Action
The result of this compound’s action is the successful synthesis of TiO2, which is useful for various strategic applications . It can also be used in the synthesis of freeze-dried titania foams, and WO3-loaded TiO2 photocatalyst applicable for the oxidation of trans-ferulic acid to vanillin .
Action Environment
The action environment significantly influences the efficacy and stability of this compound. For instance, increasing the sulfuric acid concentration up to 18 M provides a more stable TiOSO4 solution for the purpose of TiO2 formation . Furthermore, the reaction time during the hydrothermal process also impacts the concentration of titanium element in the filtrate .
Preparation Methods
Synthetic Routes and Reaction Conditions
Titanium oxysulfate can be synthesized through several methods, including the reaction of titanium dioxide with sulfuric acid. One common method involves the following steps:
Mixing Titanium Dioxide and Sulfuric Acid: Titanium dioxide (TiO₂) is mixed with concentrated sulfuric acid (H₂SO₄) to form a slurry.
Heating: The mixture is heated to initiate the reaction, resulting in the formation of this compound and water.
Filtration and Purification: The resulting solution is filtered to remove impurities and then purified to obtain this compound.
Another method involves the flotation of titanium concentrate, where titanium concentrate powder is mixed with concentrated sulfuric acid and subjected to an acid hydrolysis reaction. The mixture is then leached and filtered to obtain the this compound solution .
Industrial Production Methods
In industrial settings, this compound is often produced by the sulfate process, which involves the following steps:
Dissolution of Ilmenite Ore: Ilmenite ore (FeTiO₃) is dissolved in aqueous sulfuric acid, which precipitates the iron while retaining the titanium in solution.
Precipitation of Titanium Dioxide: The titanium sulfate solution is then diluted or heated to precipitate pure titanium dioxide (TiO₂), with this compound as an intermediate product.
Chemical Reactions Analysis
Types of Reactions
Titanium oxysulfate undergoes various chemical reactions, including:
Hydrolysis: In the presence of water, this compound hydrolyzes to form titanium dioxide and sulfuric acid.
Thermal Decomposition: Upon heating, this compound decomposes to produce titanium dioxide and sulfur oxides.
Reduction: this compound can be reduced to titanium(III) compounds under specific conditions.
Common Reagents and Conditions
Hydrolysis: Water is the primary reagent used in the hydrolysis of this compound.
Thermal Decomposition: High temperatures are required for the thermal decomposition of this compound.
Reduction: Reducing agents such as hydrogen or carbon monoxide can be used to reduce this compound.
Major Products Formed
Hydrolysis: Titanium dioxide (TiO₂) and sulfuric acid (H₂SO₄).
Thermal Decomposition: Titanium dioxide (TiO₂) and sulfur oxides (SO₂ and SO₃).
Reduction: Titanium(III) compounds and sulfur oxides.
Scientific Research Applications
Titanium oxysulfate has a wide range of scientific research applications, including:
Chemistry: It is used as a precursor for the synthesis of titanium dioxide, which is employed in photocatalysis, solar cells, and self-cleaning surfaces.
Biology: Titanium dioxide derived from this compound is used in biomedical applications such as drug delivery systems and biosensors.
Medicine: Titanium dioxide is utilized in medical implants and prosthetics due to its biocompatibility and corrosion resistance.
Comparison with Similar Compounds
Titanium oxysulfate can be compared with other titanium compounds such as:
Titanium(IV) Sulfate (Ti(SO₄)₂): Unlike this compound, titanium(IV) sulfate has a lower titanium content and is less commonly used as a precursor for titanium dioxide.
Titanium(IV) Isopropoxide (Ti(OCH(CH₃)₂)₄): This compound is used in sol-gel processes for the synthesis of titanium dioxide but differs in its reactivity and application scope.
Titanium(IV) Chloride (TiCl₄): Titanium(IV) chloride is a volatile liquid used in the production of titanium metal and titanium dioxide, but it requires different handling and reaction conditions compared to this compound.
This compound is unique due to its high titanium content and its role as an intermediate in the production of titanium dioxide, making it a valuable compound in various scientific and industrial applications.
Biological Activity
Titanium oxysulfate (TiOSO₄) is a compound that has garnered attention in various fields, particularly due to its potential biological activities. This article delves into the biological activity of this compound, examining its antimicrobial properties, synthesis methods, and applications in biomedical fields.
Overview of Titanium Oxy-sulfate
This compound is primarily used as a precursor for synthesizing titanium dioxide (TiO₂) nanoparticles, which exhibit significant biological activities. The compound is synthesized through various methods, including hydrothermal processes and chemical coprecipitation.
Antimicrobial Properties
Antimicrobial Activity : this compound has shown promising antimicrobial properties, particularly when converted into titanium dioxide nanoparticles. These nanoparticles have been observed to exhibit high efficacy against a range of microorganisms, including both Gram-positive and Gram-negative bacteria as well as fungi.
Key Findings on Antimicrobial Efficacy
- Broad Spectrum : TiO₂ nanoparticles derived from this compound demonstrate broad-spectrum antimicrobial activity. Studies indicate that these nanoparticles can effectively inhibit the growth of various pathogenic bacteria, with varying effectiveness depending on the microorganism's strain and the nanoparticle's size and morphology .
- Mechanism of Action : The antimicrobial effect is largely attributed to the generation of reactive oxygen species (ROS) upon exposure to UV light, which leads to oxidative stress in microbial cells. This mechanism has been extensively documented in literature .
- Comparative Studies : Research comparing biogenic and chemically synthesized TiO₂ nanoparticles indicates that biogenic variants often exhibit enhanced antibacterial properties due to the stabilizing effects of plant extracts used during synthesis .
Data Table: Antimicrobial Efficacy of TiO₂ Nanoparticles
Microorganism Type | Inhibition Zone Diameter (mm) | Reference |
---|---|---|
Gram-positive (e.g., Staphylococcus aureus) | 15-20 | |
Gram-negative (e.g., Escherichia coli) | 18-25 | |
Fungi (e.g., Candida albicans) | 10-15 |
Synthesis Methods
Various synthesis methods for this compound have been explored, impacting the biological activity of the resultant nanoparticles.
Hydrothermal Synthesis
The hydrothermal method involves treating ilmenite with sulfuric acid to yield this compound, which is then processed to produce TiO₂. This method has shown promise in producing nanoparticles with desirable characteristics for biomedical applications .
Chemical Coprecipitation
This method allows for the simultaneous precipitation of this compound with other compounds, facilitating the formation of composite materials that may enhance biological activity. The coprecipitation technique has been utilized to create hybrid materials with improved stability and functionality .
Case Study 1: Antibacterial Activity Assessment
A study assessed the antibacterial activity of TiO₂ nanoparticles synthesized from this compound using Hibiscus flower extract. Results indicated that these biogenic nanoparticles exhibited superior antibacterial properties compared to their chemically synthesized counterparts, highlighting the role of natural stabilizers in enhancing efficacy .
Case Study 2: Detection Applications
Research demonstrated that titanium(IV) oxysulfate can be employed in gas-phase detection methods for hydrogen peroxide with high sensitivity. This application underscores the versatility of this compound beyond its antimicrobial properties, suggesting potential uses in environmental monitoring and safety applications .
Properties
CAS No. |
123334-00-9 |
---|---|
Molecular Formula |
O5STi |
Molecular Weight |
159.93 |
Origin of Product |
United States |
Disclaimer and Information on In-Vitro Research Products
Please be aware that all articles and product information presented on BenchChem are intended solely for informational purposes. The products available for purchase on BenchChem are specifically designed for in-vitro studies, which are conducted outside of living organisms. In-vitro studies, derived from the Latin term "in glass," involve experiments performed in controlled laboratory settings using cells or tissues. It is important to note that these products are not categorized as medicines or drugs, and they have not received approval from the FDA for the prevention, treatment, or cure of any medical condition, ailment, or disease. We must emphasize that any form of bodily introduction of these products into humans or animals is strictly prohibited by law. It is essential to adhere to these guidelines to ensure compliance with legal and ethical standards in research and experimentation.