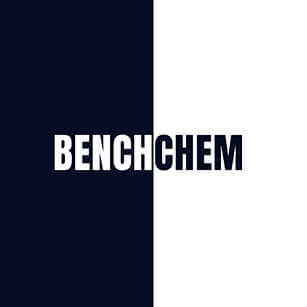
8-AZIDOADENOSINE-5/'-O-DIPHOSPHATE SODIUM SALT
- Click on QUICK INQUIRY to receive a quote from our team of experts.
- With the quality product at a COMPETITIVE price, you can focus more on your research.
Overview
Description
8-Azidoadenosine-5’-O-diphosphate sodium salt is a chemical compound with the molecular formula C10H13N8O10P2Na. It is commonly used as a photo-affinity probe in biochemical research. This compound is known for its ability to form covalent bonds with target proteins upon activation by long-wavelength ultraviolet light, making it a valuable tool in the study of protein interactions and functions .
Mechanism of Action
Target of Action
The primary target of 8-Azidoadenosine-5’-O-Diphosphate Sodium Salt, also known as 8-azido-ADP, is the ATP precursor and purinergic receptor ligand ADP . It has been used as a photoaffinity label for various proteins, including glutamate dehydrogenase (GDH) isoproteins isolated from bovine brains, as well as eukaryotic translation initiation factor 2 (eIF2) and guanine nucleotide exchange factor (GEF) isolated from rabbit reticulocytes .
Mode of Action
8-azido-ADP is a clickable form of ADP . It is potentially utilized as a photo-affinity probe commonly in combination with 32P . Long wavelength UV light activates the Azido group which then creates a nitrene moiety . This reactive nitrene is then able to covalently bond with residues of target proteins .
Biochemical Pathways
The biochemical pathways affected by 8-azido-ADP are those involving the ATP precursor and purinergic receptor ligand ADP . The compound’s interaction with these targets can influence the function of various proteins, including GDH isoproteins, eIF2, and GEF .
Pharmacokinetics
It is known that the compound is soluble in water , which could potentially influence its absorption, distribution, metabolism, and excretion (ADME) properties.
Result of Action
The result of 8-azido-ADP’s action is the covalent bonding with residues of target proteins . This can lead to changes in the function of these proteins, potentially affecting cellular processes such as energy metabolism (in the case of ATP precursor and purinergic receptor ligand ADP) and protein synthesis (in the case of eIF2 and GEF) .
Action Environment
The action of 8-azido-ADP can be influenced by environmental factors such as the presence of UV light, which is necessary to activate the Azido group
Biochemical Analysis
Biochemical Properties
8-Azidoadenosine-5’-O-diphosphate Sodium Salt interacts with various enzymes and proteins. Radiolabeled forms of 8-azido-ADP have been used as photoaffinity labels for proteins such as glutamate dehydrogenase (GDH) isoproteins isolated from bovine brains, as well as eukaryotic translation initiation factor 2 (eIF2) and guanine nucleotide exchange factor (GEF) isolated from rabbit reticulocytes .
Cellular Effects
The effects of 8-Azidoadenosine-5’-O-diphosphate Sodium Salt on cellular processes are primarily through its interactions with proteins and enzymes. It influences cell function by acting as a photoaffinity label, allowing for the study of protein interactions and functions .
Molecular Mechanism
At the molecular level, 8-Azidoadenosine-5’-O-diphosphate Sodium Salt exerts its effects through binding interactions with biomolecules. It acts as a photoaffinity label, binding to proteins such as GDH isoproteins, eIF2, and GEF .
Preparation Methods
Synthetic Routes and Reaction Conditions
The synthesis of 8-Azidoadenosine-5’-O-diphosphate sodium salt typically involves the introduction of an azido group to adenosine-5’-O-diphosphate. This can be achieved through a series of chemical reactions, including nucleophilic substitution and phosphorylation. The reaction conditions often require the use of specific reagents and catalysts to ensure the successful incorporation of the azido group .
Industrial Production Methods
Chemical Reactions Analysis
Types of Reactions
8-Azidoadenosine-5’-O-diphosphate sodium salt undergoes various chemical reactions, including:
Photoactivation: Upon exposure to long-wavelength ultraviolet light, the azido group is activated to form a highly reactive nitrene moiety.
Covalent Bond Formation: The reactive nitrene can form covalent bonds with residues of target proteins, facilitating the study of protein interactions.
Common Reagents and Conditions
Ultraviolet Light: Used to activate the azido group.
Buffer Solutions: Maintain the pH and ionic strength required for the reactions.
Target Proteins: The compound interacts with specific proteins to form covalent bonds.
Major Products Formed
The primary product formed from the reactions involving 8-Azidoadenosine-5’-O-diphosphate sodium salt is a covalently bonded complex between the compound and the target protein. This complex can be analyzed to gain insights into protein structure and function .
Scientific Research Applications
8-Azidoadenosine-5’-O-diphosphate sodium salt has a wide range of applications in scientific research, including:
Comparison with Similar Compounds
Similar Compounds
8-Azidoadenosine-5’-triphosphate: Another photo-affinity probe with similar applications in biochemical research.
8-Azidoadenosine-5’-monophosphate: Used in studies of nucleotide-protein interactions.
Uniqueness
8-Azidoadenosine-5’-O-diphosphate sodium salt is unique in its ability to form covalent bonds with target proteins upon photoactivation. This property makes it a valuable tool for studying protein interactions and functions, distinguishing it from other similar compounds .
Biological Activity
8-Azidoadenosine-5'-O-diphosphate sodium salt (8-N3-ADP) is a modified nucleotide that plays a significant role in biochemical research, particularly in the study of purinergic signaling and receptor interactions. Its unique azido group allows for photoaffinity labeling and click chemistry applications, making it a valuable tool in molecular biology and pharmacology.
- Chemical Formula : C10H14N8O10P2
- Molecular Weight : 468.2 g/mol
- Purity : >95% (HPLC)
- Absorption Maximum : λmax 281 nm
- Solubility : Soluble in water at pH 7.6
8-N3-ADP acts as a competitive inhibitor of various enzymes involved in nucleotide metabolism. Its azido group allows for covalent bonding to target proteins upon exposure to UV light, facilitating the study of protein interactions and functions.
Key Mechanisms:
- Photoaffinity Labeling : The azido group can form stable bonds with nucleophilic residues in proteins, allowing researchers to identify and characterize ADP-binding proteins.
- Binding Affinity : Studies indicate that 8-N3-ADP exhibits similar binding affinities to purinergic receptors compared to its non-modified counterparts, suggesting that the azido modification does not significantly alter its interaction with these proteins .
1. Interaction with P-glycoprotein (Pgp)
Research has demonstrated that 8-N3-ADP can trap transition states of Pgp, a crucial ATP-dependent drug efflux pump implicated in multidrug resistance in cancer cells. The trapping occurs even in the absence of ATP hydrolysis, indicating that both ATP sites on Pgp can interact with 8-N3-ADP effectively .
Parameter | Value |
---|---|
Energy of Activation | 152 kJ/mol |
Half-maximal Trapping Concentration | Similar for 8-N3-ATP and 8-N3-ADP |
2. Modulation of ADP-Binding Proteins
8-N3-ADP is recognized as a modulator of ADP-binding proteins, enhancing metabolic stability and providing insights into the stereospecificity of ADP-responsive receptors . This property is particularly useful for characterizing receptor-ligand interactions in various biological systems.
3. Antiviral Activity
While primarily studied for its effects on purinergic signaling, there is potential for 8-N3-ADP to exhibit antiviral properties similar to other nucleoside analogs. These compounds often act as competitive inhibitors for viral enzymes, which could lead to their incorporation into viral RNA or DNA, ultimately disrupting viral replication processes .
Case Study 1: Photoaffinity Labeling in Cancer Research
A study utilized 8-N3-ADP to identify ADP-binding proteins involved in drug resistance mechanisms in cancer cells. By employing UV irradiation, researchers were able to covalently label these proteins, facilitating their subsequent isolation and characterization.
Case Study 2: Interaction with Antiviral Targets
In another investigation, 8-N3-ADP was tested against various viral enzymes to evaluate its potential as an antiviral agent. The results indicated that while it did not exhibit direct antiviral activity, its structural analogs showed promise when incorporated into viral genomes, leading to chain termination during replication .
Properties
CAS No. |
102185-14-8 |
---|---|
Molecular Formula |
C10H13N8NaO10P2 |
Molecular Weight |
490.2 |
Origin of Product |
United States |
Disclaimer and Information on In-Vitro Research Products
Please be aware that all articles and product information presented on BenchChem are intended solely for informational purposes. The products available for purchase on BenchChem are specifically designed for in-vitro studies, which are conducted outside of living organisms. In-vitro studies, derived from the Latin term "in glass," involve experiments performed in controlled laboratory settings using cells or tissues. It is important to note that these products are not categorized as medicines or drugs, and they have not received approval from the FDA for the prevention, treatment, or cure of any medical condition, ailment, or disease. We must emphasize that any form of bodily introduction of these products into humans or animals is strictly prohibited by law. It is essential to adhere to these guidelines to ensure compliance with legal and ethical standards in research and experimentation.