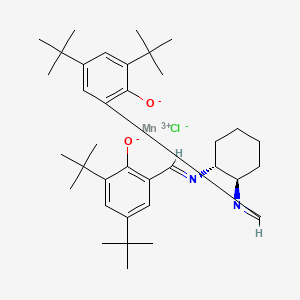
(R,R)-Jacobsen's catalyst
- Click on QUICK INQUIRY to receive a quote from our team of experts.
- With the quality product at a COMPETITIVE price, you can focus more on your research.
Overview
Description
(R,R)-Jacobsen’s catalyst is a chiral manganese(III) salen complex that has gained significant attention in the field of asymmetric catalysis. This compound is known for its ability to catalyze a variety of enantioselective reactions, making it a valuable tool in organic synthesis. The catalyst is named after its discoverer, Eric Jacobsen, who developed it in the early 1990s.
Mechanism of Action
Target of Action
The primary targets of the (R,R)-Jacobsen’s catalyst are unsaturated hydrocarbons, imines, nitro, and carbonyl compounds . The catalyst is particularly effective in promoting transfer hydrogenation (TH) and asymmetric transfer hydrogenation (ATH) reactions of these compounds . These reactions are crucial in organic synthesis, with applications ranging from the synthesis of fine chemicals to pharmaceuticals .
Mode of Action
The (R,R)-Jacobsen’s catalyst interacts with its targets through a process known as catalysis . In a typical catalytic mechanism, the catalyst forms an intermediate with one of the reactants, which opens up a reaction path for a quick transformation . After this, the catalyst is released and can form another reactive intermediate . This process reduces the activation energy of the reaction, thereby increasing the reaction rate .
Biochemical Pathways
The (R,R)-Jacobsen’s catalyst affects the biochemical pathways involved in the metabolism of unsaturated hydrocarbons, imines, nitro, and carbonyl compounds . By promoting TH and ATH reactions, the catalyst facilitates the conversion of these compounds into more stable or useful forms . The downstream effects of these reactions can include the production of various fine chemicals and pharmaceuticals .
Pharmacokinetics
It’s worth noting that the catalyst’s effectiveness can be influenced by its physical and chemical properties . For instance, its rigidity, lipophilicity, steric bulkiness, and electron richness can affect its bioavailability and its interaction with target compounds .
Result of Action
The molecular and cellular effects of the (R,R)-Jacobsen’s catalyst’s action primarily involve the transformation of target compounds. By promoting TH and ATH reactions, the catalyst facilitates the conversion of unsaturated hydrocarbons, imines, nitro, and carbonyl compounds into more stable or useful forms . This can lead to the production of various fine chemicals and pharmaceuticals .
Action Environment
The action, efficacy, and stability of the (R,R)-Jacobsen’s catalyst can be influenced by various environmental factors. For instance, the solvent used in the catalytic process can have a significant impact on the catalyst’s performance . Solvents interact directly with the catalyst, the substrates, and the products, and these interactions can increase or decrease the reaction rate and/or selectivity . Therefore, choosing the right solvent for a catalytic process can improve the outcome of the reaction considerably .
Preparation Methods
Synthetic Routes and Reaction Conditions
The synthesis of (R,R)-Jacobsen’s catalyst typically involves the condensation of a chiral diamine with salicylaldehyde, followed by complexation with manganese(III) acetate. The reaction is carried out under an inert atmosphere to prevent oxidation of the manganese center. The general steps are as follows:
Condensation Reaction: The chiral diamine is reacted with salicylaldehyde in the presence of a base to form the salen ligand.
Complexation: The salen ligand is then reacted with manganese(III) acetate in a suitable solvent, such as ethanol or methanol, to form the (R,R)-Jacobsen’s catalyst.
Industrial Production Methods
Industrial production of (R,R)-Jacobsen’s catalyst follows similar synthetic routes but on a larger scale. The process involves careful control of reaction conditions to ensure high yield and purity of the catalyst. The use of automated systems and continuous flow reactors can enhance the efficiency and scalability of the production process.
Chemical Reactions Analysis
Types of Reactions
(R,R)-Jacobsen’s catalyst is primarily used in oxidation reactions, particularly in the asymmetric epoxidation of alkenes. It can also catalyze other types of reactions, such as:
Oxidation: Epoxidation of alkenes using oxidants like sodium hypochlorite or iodosylbenzene.
Reduction: Although less common, it can be used in certain reduction reactions.
Substitution: It can facilitate nucleophilic substitution reactions in specific cases.
Common Reagents and Conditions
Oxidants: Sodium hypochlorite, iodosylbenzene, and hydrogen peroxide.
Solvents: Dichloromethane, ethanol, and methanol.
Conditions: Reactions are typically carried out at room temperature or slightly elevated temperatures, under an inert atmosphere to prevent oxidation of the catalyst.
Major Products
The major products of reactions catalyzed by (R,R)-Jacobsen’s catalyst are often enantiomerically enriched epoxides, which are valuable intermediates in the synthesis of pharmaceuticals and fine chemicals.
Scientific Research Applications
Chemistry
In chemistry, (R,R)-Jacobsen’s catalyst is widely used for the asymmetric epoxidation of alkenes, which is a crucial step in the synthesis of many chiral compounds. Its ability to produce enantiomerically pure products makes it a valuable tool in the development of new drugs and materials.
Biology and Medicine
In biology and medicine, the catalyst is used to synthesize chiral intermediates for pharmaceuticals. Its application in the production of enantiomerically pure compounds is essential for the development of drugs with specific biological activities.
Industry
In the industrial sector, (R,R)-Jacobsen’s catalyst is employed in the large-scale production of fine chemicals and pharmaceuticals. Its efficiency and selectivity make it an attractive option for processes that require high enantiomeric purity.
Comparison with Similar Compounds
Similar Compounds
Sharpless Epoxidation Catalyst: Another widely used catalyst for asymmetric epoxidation, but it uses titanium and tartrate ligands.
Katsuki-Jacobsen Catalyst: A similar manganese-based catalyst but with different chiral ligands.
Ruthenium-Based Catalysts: Used for asymmetric hydrogenation and transfer hydrogenation reactions.
Uniqueness
(R,R)-Jacobsen’s catalyst is unique in its ability to provide high enantioselectivity in epoxidation reactions. Its chiral salen ligand creates a highly selective environment, making it superior to many other catalysts in terms of enantiomeric excess and yield.
Properties
CAS No. |
138124-32-0 |
---|---|
Molecular Formula |
C36H54ClMnN2O2- |
Molecular Weight |
637.2 g/mol |
IUPAC Name |
2,4-ditert-butyl-6-[[(1R,2R)-2-[(3,5-ditert-butyl-2-hydroxyphenyl)methylideneamino]cyclohexyl]iminomethyl]phenol;manganese;chloride |
InChI |
InChI=1S/C36H54N2O2.ClH.Mn/c1-33(2,3)25-17-23(31(39)27(19-25)35(7,8)9)21-37-29-15-13-14-16-30(29)38-22-24-18-26(34(4,5)6)20-28(32(24)40)36(10,11)12;;/h17-22,29-30,39-40H,13-16H2,1-12H3;1H;/p-1/t29-,30-;;/m1../s1 |
InChI Key |
FUUKZEVIXZEIBV-SEILFYAJSA-M |
SMILES |
CC(C)(C)C1=CC(=C(C(=C1)C(C)(C)C)[O-])C=NC2CCCCC2N=CC3=C(C(=CC(=C3)C(C)(C)C)C(C)(C)C)[O-].[Cl-].[Mn+3] |
Isomeric SMILES |
CC(C)(C)C1=CC(=C(C(=C1)C(C)(C)C)O)C=N[C@@H]2CCCC[C@H]2N=CC3=C(C(=CC(=C3)C(C)(C)C)C(C)(C)C)O.[Cl-].[Mn] |
Canonical SMILES |
CC(C)(C)C1=CC(=C(C(=C1)C(C)(C)C)O)C=NC2CCCCC2N=CC3=C(C(=CC(=C3)C(C)(C)C)C(C)(C)C)O.[Cl-].[Mn] |
physical_description |
Dark brown powder; [Sigma-Aldrich MSDS] |
Synonyms |
Jacobsen’s Catalyst; Chloro[[2,2’-[(1R,2R)-1,2-cyclohexanediylbis[(nitrilo-κN)methylidyne]]bis[4,6-bis(1,1-dimethylethyl)phenolato-κO]](2-)]manganese; (-)-Chloro{(1R,2R)-4,4’,6,6’-tetra-tert-butyl-2,2’-[cyclohexane-1,2-diylbis(nitrilomethylidyne)]dip |
Origin of Product |
United States |
Q1: What is the molecular formula and weight of Jacobsen's catalyst?
A1: The molecular formula of Jacobsen's catalyst is C36H54ClMnN2O2, and its molecular weight is 623.24 g/mol.
Q2: Is there spectroscopic data available for Jacobsen's catalyst?
A2: Yes, Jacobsen's catalyst has been characterized by various spectroscopic techniques, including: * UV-Vis Spectroscopy: [] [] This technique reveals characteristic electronic transitions for the catalyst.* Infrared Spectroscopy (IR): [] [] This technique identifies characteristic functional groups like the imine group (HC=N).* Nuclear Magnetic Resonance (NMR): [] [] [] While less informative for the paramagnetic Mn(III) complex, NMR is valuable for characterizing the ligand and diamagnetic derivatives. * Circular Dichroism (CD): [] [] CD provides information about the chiral environment of the manganese center and ligand conformation.
Q3: What is the primary application of Jacobsen's catalyst?
A3: Jacobsen's catalyst is widely recognized for its role in the enantioselective epoxidation of unfunctionalized olefins. [] [] This means it catalyzes the formation of epoxides from alkenes with high selectivity for a specific enantiomer, a crucial aspect in pharmaceutical and fine chemical synthesis.
Q4: What oxidants are typically used with Jacobsen's catalyst in epoxidation reactions?
A4: Common oxidants employed with Jacobsen's catalyst include:* Sodium hypochlorite (NaOCl) [] []* meta-Chloroperoxybenzoic acid (m-CPBA) [] [] [] []* Iodosylbenzene (PhIO) [] [] []* Hydrogen peroxide (H2O2) [] []
Q5: How does the structure of the Jacobsen's catalyst contribute to its enantioselectivity?
A5: The chirality of Jacobsen's catalyst originates from the (R,R)-cyclohexane-1,2-diamine backbone of the salen ligand. This creates a chiral environment around the manganese center, favoring the approach and reaction of one enantiomer of the olefin substrate over the other. [] [] []
Q6: What are the limitations of Jacobsen's catalyst in epoxidation reactions?
A6: Some limitations of Jacobsen's catalyst include:* Susceptibility to oxidative degradation: The catalyst can undergo degradation under oxidative conditions, particularly at the imine groups of the salen ligand, leading to decreased activity upon reuse. [] []* Formation of inactive dimeric species: Under certain conditions, Jacobsen's catalyst can form inactive μ-oxo-manganese(IV) dimers, reducing its catalytic efficiency. []* Dependence on axial ligands: The activity and enantioselectivity of Jacobsen's catalyst can be influenced by the presence and nature of axial ligands. [] []
Q7: Are there any alternative applications of Jacobsen's catalyst?
A7: While primarily known for epoxidation, Jacobsen's catalyst has also been explored for other reactions, including:* Hydrolytic kinetic resolution of epoxides: [] This process uses water as a nucleophile to open an epoxide ring, selectively producing one enantiomer of the corresponding diol.* Sulfoxidation, chlorination, and hydrogen abstraction reactions: [] While less commonly employed, the bis-hypochlorite adduct of Jacobsen's catalyst has shown activity in these transformations.
Q8: Can Jacobsen's catalyst be immobilized on solid supports?
A8: Yes, immobilization of Jacobsen's catalyst on various solid supports has been extensively investigated to enhance stability and enable recycling. [] [] [] [] [] [] Commonly employed supports include:* Mesoporous silica materials like SBA-15 and MCM-41: These materials offer high surface area and tunable pore sizes.* Polymeric resins: Polystyrene and polymethacrylate-based resins have been used for catalyst immobilization.* Zirconium phosphate-based materials: These materials provide unique properties for catalyst anchoring.
Q9: What are the benefits and drawbacks of immobilizing Jacobsen's catalyst?
A9: Benefits of immobilization include:* Improved stability: Immobilization can physically separate catalyst molecules, hindering the formation of inactive dimers and potentially improving stability. []* Facilitated catalyst recovery and recycling: Immobilized catalysts can be easily separated from the reaction mixture and reused, improving process efficiency and cost-effectiveness. []
Q10: Have computational methods been used to study Jacobsen's catalyst?
A10: Yes, computational chemistry, particularly density functional theory (DFT) calculations, has been employed to:* Investigate the mechanism of oxygen transfer: These studies provide insights into the intermediates and transition states involved in the epoxidation reaction. []* Study the influence of axial ligands on catalyst activity: Calculations can help rationalize the role of axial ligands in modulating the electronic properties and reactivity of the catalyst. []* Explore the impact of ligand modifications on enantioselectivity: DFT calculations can be used to predict the impact of structural changes to the salen ligand on the enantioselectivity of the catalyst. []
Q11: How do modifications to the salen ligand affect the catalytic properties of Jacobsen's catalyst?
A11: Modifications to the salen ligand can significantly influence the catalyst's performance:* Substituents on the aromatic rings: Altering the electronic properties of the aromatic rings by introducing electron-donating or electron-withdrawing groups can impact the catalyst's activity and selectivity. [] []* Chirality of the diamine backbone: The configuration of the diamine backbone determines the absolute configuration of the epoxide product. Different diamine backbones can lead to variations in enantioselectivity. [] [] []* Linker rigidity in immobilized systems: The rigidity of the linker used to attach the catalyst to a solid support can influence the catalyst's activity and selectivity. Rigid linkers often lead to better performance compared to flexible ones. []
Q12: What were the key milestones in the development and application of Jacobsen's catalyst?
A12:
Early 1990s: Eric Jacobsen and coworkers first reported the use of chiral Mn(III) salen complexes, including Jacobsen's catalyst, for enantioselective epoxidation reactions. This discovery revolutionized asymmetric catalysis and earned Jacobsen the 2018 Arthur C. Cope Award. []* Subsequent decades: Extensive research has focused on understanding the mechanism of action, expanding the substrate scope, and developing strategies to overcome the limitations of Jacobsen's catalyst. These efforts have led to the development of numerous modified catalysts and immobilization strategies. [] [] [] [] * Present:* Jacobsen's catalyst remains a benchmark catalyst for asymmetric epoxidation and continues to inspire the development of new chiral catalysts for various asymmetric transformations. []
Disclaimer and Information on In-Vitro Research Products
Please be aware that all articles and product information presented on BenchChem are intended solely for informational purposes. The products available for purchase on BenchChem are specifically designed for in-vitro studies, which are conducted outside of living organisms. In-vitro studies, derived from the Latin term "in glass," involve experiments performed in controlled laboratory settings using cells or tissues. It is important to note that these products are not categorized as medicines or drugs, and they have not received approval from the FDA for the prevention, treatment, or cure of any medical condition, ailment, or disease. We must emphasize that any form of bodily introduction of these products into humans or animals is strictly prohibited by law. It is essential to adhere to these guidelines to ensure compliance with legal and ethical standards in research and experimentation.