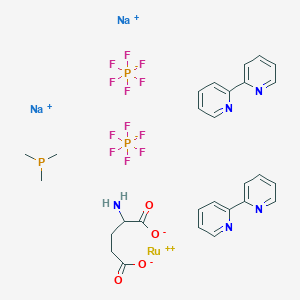
RuBi-Glutamate
- Click on QUICK INQUIRY to receive a quote from our team of experts.
- With the quality product at a COMPETITIVE price, you can focus more on your research.
Overview
Description
RuBi-Glutamate is a novel caged-glutamate compound based on ruthenium photochemistry. It is composed of a ruthenium center with six coordination positions, four of which are occupied by two bidentate bipyridines, the fifth by a trimethylphosphine, and the sixth by a molecule of L-glutamate . This compound can be excited with visible wavelengths and releases glutamate after one- or two-photon excitation . This compound is known for its high quantum efficiency and can be used at low concentrations, partly avoiding the blockade of GABAergic transmission present with other caged compounds .
Preparation Methods
The synthesis of RuBi-Glutamate involves the coordination of L-glutamate to a ruthenium center. The synthetic route typically includes the following steps:
Preparation of Ruthenium Complex: The ruthenium complex is prepared by reacting ruthenium trichloride with bipyridine and trimethylphosphine ligands.
Coordination of L-Glutamate: The L-glutamate is then coordinated to the ruthenium center through its amino group.
Chemical Reactions Analysis
RuBi-Glutamate undergoes photochemical reactions upon exposure to light. The primary reaction is the release of glutamate from the ruthenium center upon absorption of one photon of visible light or two near-infrared photons . This process involves the excitation of electrons in the ruthenium complex, leading to a rearrangement of bonds and the release of glutamate. The exact chemical equation for this process is complex and involves excited electronic states.
Scientific Research Applications
Key Applications in Neuroscience
- Neuronal Activation :
-
Studying Synaptic Transmission :
- The compound can be used to investigate synaptic currents in various settings. For instance, voltage-clamp recordings have shown that this compound activates glutamate receptors in cortical pyramidal neurons, generating excitatory postsynaptic currents (EPSCs) without significantly affecting spontaneous excitatory synaptic transmission .
- Functional Mapping :
- Reduction of Non-specific Effects :
Case Study 1: Two-Photon Uncaging
In a study examining the efficacy of this compound for two-photon uncaging, researchers found that using an excitation wavelength of 800 nm and a concentration of 300 μM produced reliable excitatory responses in layer 2/3 pyramidal neurons. The amplitude of uncaging-induced depolarizations ranged from 1 to 20 mV, confirming its effectiveness in activating glutamate receptors .
Case Study 2: Impact on Inhibitory Synaptic Transmission
Another investigation focused on the effects of this compound on inhibitory postsynaptic currents (IPSCs). While bath application of 300 μM this compound showed a trend towards reducing the amplitude and frequency of spontaneous IPSCs, only the reduction in frequency was statistically significant when sodium channel blockers were applied .
Data Tables
Parameter | Observation |
---|---|
Excitation Wavelength | Optimal at 800 nm |
Effective Concentration | 300 μM for reliable responses |
Depolarization Amplitude | Ranges from 1 to 20 mV |
Effect on EPSCs | Minimal impact on spontaneous EPSCs |
Effect on IPSCs | Reduction in frequency observed under specific conditions |
Mechanism of Action
The mechanism of action of RuBi-Glutamate involves the photorelease of glutamate upon exposure to light. When this compound absorbs one photon of visible light or two near-infrared photons, it photocleaves within nanoseconds, releasing glutamate from the ruthenium-bipyridine core . This process has a quantum yield of approximately 0.13 at pH 7 and an extinction coefficient of over 4,000 M−1 cm−1 at 473 nm . The released glutamate then acts on glutamate receptors in neurons, leading to excitatory responses .
Comparison with Similar Compounds
RuBi-Glutamate is unique compared to other caged-glutamate compounds due to its ability to be excited with visible wavelengths and its high quantum efficiency . Similar compounds include:
MNI-Glutamate: Requires higher concentrations for effective two-photon uncaging and has more nonspecific effects.
CDNI-Glutamate: Exhibits a higher two-photon cross-section but requires UV light for activation.
DNI-Glutamate: Similar to CDNI-Glutamate but with different photophysical properties.
This compound’s ability to release glutamate with visible light and its reduced nonspecific effects make it a superior choice for certain neurobiological applications .
Biological Activity
RuBi-Glutamate is a novel caged glutamate compound characterized by its ability to release glutamate upon excitation with visible light or through two-photon activation. This compound is particularly significant in neurobiological research due to its high quantum efficiency and minimal interference with GABAergic transmission, making it a valuable tool for studying synaptic mechanisms and neuronal circuits.
- Chemical Name : (bis(2,2'-Bipyridine-N,N')trimethylphosphine)-(S)-1-aminopropane-1,3-dicarboxylic acid ruthenium(2+) complex sodium hexafluorophosphate salt
- Molecular Weight : 970.54 g/mol
- Molecular Formula : C28H32N5O4PRu.2NaPF6
- CAS Number : 2417096-44-5
- Appearance : Orange solid, soluble in water (20 mM) .
This compound operates by undergoing photolysis when exposed to specific wavelengths of light, resulting in the release of glutamate. This process can be initiated through:
- One-photon excitation : Typically using visible light.
- Two-photon excitation : Utilizing near-infrared light, which allows for deeper tissue penetration and reduced scattering .
1. Excitatory Responses
This compound has been shown to generate excitatory postsynaptic currents (EPSCs) in pyramidal neurons within the neocortex. When applied at a concentration of 300 μM, it effectively activates glutamate receptors without significantly affecting spontaneous synaptic currents .
2. Spatial Resolution
The compound enables high spatial resolution in neuronal activation, allowing researchers to target individual dendritic spines with precision. This capability is crucial for studying synaptic plasticity and the dynamics of neurotransmitter release .
3. GABAergic Transmission
Unlike other caged glutamates, this compound minimizes the blockade of GABAergic transmission. Studies indicate that it does not significantly alter the amplitude or frequency of inhibitory postsynaptic currents (IPSCs) at concentrations that effectively activate excitatory synapses .
Study 1: Two-photon Activation in Neurons
In a study published in Neuron, researchers utilized two-photon uncaging of this compound to stimulate layer 2/3 pyramidal neurons. The results demonstrated reliable action potential generation with minimal interference from inhibitory signaling pathways .
Study 2: Network Activity Modulation
Research highlighted in Nature explored how this compound could modulate network-level activity in cortical astrocytes. The study found that brief glutamate inputs led to significant calcium responses, indicating the compound's potential in examining astrocytic roles in neurotransmission .
Data Tables
Property | Value |
---|---|
Chemical Structure | Chemical Structure |
Optimal Excitation Wavelength | 800 nm |
Concentration for Effective Activation | 300 μM |
Quantum Efficiency | High |
Solubility | Water-soluble (20 mM) |
Q & A
Basic Research Questions
Q. How does RuBi-Glutamate enable precise spatiotemporal control of neuronal activation in experimental settings?
this compound is a "caged" glutamate compound where glutamate is bound to a photolabile protecting group. Upon irradiation with visible or two-photon laser light (e.g., 450 nm or 720–900 nm), the bond breaks, releasing bioactive glutamate . This allows researchers to activate neurons with micrometer-scale spatial resolution by targeting specific dendritic regions or synapses. For methodological rigor, calibrate light intensity and duration to avoid overstimulation or cytotoxicity. Use electrophysiological recordings (e.g., patch-clamp) to validate glutamate release efficacy and postsynaptic responses .
Q. What are the critical parameters to optimize when using this compound in in vitro neural circuit studies?
Key parameters include:
- Light wavelength and power : Ensure compatibility with two-photon microscopy systems (e.g., 720–900 nm for deep-tissue penetration) .
- Concentration : Typical working concentrations range from 1–5 mM in physiological buffers; higher concentrations risk nonspecific activation .
- Temporal resolution : Pulse durations of 1–10 ms achieve millisecond-scale glutamate release, mimicking physiological synaptic transmission . Include control experiments with light-only stimulation to rule out photothermal artifacts .
Advanced Research Questions
Q. How can researchers optimize this compound concentration to balance neuronal activation efficacy and cytotoxicity in chronic imaging studies?
Cytotoxicity arises from prolonged light exposure or excessive glutamate release. Conduct dose-response experiments:
- Titrate this compound (0.5–10 mM) while monitoring cell viability via markers like propidium iodide or lactate dehydrogenase (LDH) assays .
- Pair with calcium imaging (e.g., GCaMP) to correlate glutamate release with neuronal activity without overstimulation .
- Use lower concentrations (1–2 mM) for longitudinal studies to minimize oxidative stress .
Q. What experimental strategies resolve conflicting data on this compound’s spatial resolution in dense neural networks?
Contradictions may stem from differences in:
- Laser focal volume : Smaller focal spots (e.g., 2–5 µm) improve precision but reduce signal-to-noise ratio. Validate with point-spread function measurements .
- Uncaging efficiency : Quantify using fluorescent glutamate analogs (e.g., MNI-glutamate-fluorescein) to map release zones .
- Network feedback : Inhibitory interneurons may suppress downstream activity; combine this compound with optogenetic silencing (e.g., ArchT) to isolate direct effects .
Q. How should researchers address variability in this compound’s temporal resolution across different experimental models (e.g., slice vs. in vivo)?
Variability arises from tissue scattering, depth, and perfusion dynamics. Methodological solutions include:
- Slice preparation : Use acute slices <300 µm thick to ensure adequate oxygen and drug diffusion .
- In vivo calibration : Pre-calibrate light power using a cranial window and multiphoton raster scanning to account for scattering .
- Pharmacological controls : Apply glutamate receptor antagonists (e.g., CNQX/AP5) to confirm this compound-specific effects .
Q. Methodological Best Practices
Q. What controls are essential for validating this compound-specific effects in synaptic plasticity experiments?
- Light-only controls : Irradiate the sample without this compound to exclude phototoxicity .
- Pharmacological blockade : Use NMDA/AMPA receptor antagonists to confirm glutamate-mediated responses .
- Caged inert compounds : Test analogues like RuBi-GABA to rule out nonspecific caged-molecule effects .
Q. How can researchers integrate this compound with simultaneous electrophysiology and calcium imaging?
- Optrode design : Use quartz electrodes or transparent graphene-based probes to minimize light scattering .
- Synchronization : Trigger light pulses and data acquisition via a Master-9 stimulator or similar device to align uncaging with recording .
- Post-hoc analysis : Employ custom scripts (e.g., Python/Matlab) to deconvolve calcium transients from uncaging artifacts .
Q. Data Analysis and Interpretation
Q. What statistical approaches are recommended for analyzing this compound-evoked network dynamics?
- Cross-correlation analysis : Identify time-locked interactions between stimulated and downstream neurons .
- Graph theory metrics : Calculate node centrality or clustering coefficients to map network connectivity changes .
- Machine learning : Train classifiers (e.g., SVM) to distinguish direct vs. polysynaptic responses based on latency and amplitude .
Q. How can researchers reconcile discrepancies between this compound data and traditional microiontophoresis results?
Differences may reflect:
Properties
IUPAC Name |
disodium;2-aminopentanedioate;2-pyridin-2-ylpyridine;ruthenium(2+);trimethylphosphane;dihexafluorophosphate |
Source
|
---|---|---|
Source | PubChem | |
URL | https://pubchem.ncbi.nlm.nih.gov | |
Description | Data deposited in or computed by PubChem | |
InChI |
InChI=1S/2C10H8N2.C5H9NO4.C3H9P.2F6P.2Na.Ru/c2*1-3-7-11-9(5-1)10-6-2-4-8-12-10;6-3(5(9)10)1-2-4(7)8;1-4(2)3;2*1-7(2,3,4,5)6;;;/h2*1-8H;3H,1-2,6H2,(H,7,8)(H,9,10);1-3H3;;;;;/q;;;;2*-1;2*+1;+2/p-2 |
Source
|
Source | PubChem | |
URL | https://pubchem.ncbi.nlm.nih.gov | |
Description | Data deposited in or computed by PubChem | |
InChI Key |
SQQVSHWHIPVYSZ-UHFFFAOYSA-L |
Source
|
Source | PubChem | |
URL | https://pubchem.ncbi.nlm.nih.gov | |
Description | Data deposited in or computed by PubChem | |
Canonical SMILES |
CP(C)C.C1=CC=NC(=C1)C2=CC=CC=N2.C1=CC=NC(=C1)C2=CC=CC=N2.C(CC(=O)[O-])C(C(=O)[O-])N.F[P-](F)(F)(F)(F)F.F[P-](F)(F)(F)(F)F.[Na+].[Na+].[Ru+2] |
Source
|
Source | PubChem | |
URL | https://pubchem.ncbi.nlm.nih.gov | |
Description | Data deposited in or computed by PubChem | |
Molecular Formula |
C28H32F12N5Na2O4P3Ru |
Source
|
Source | PubChem | |
URL | https://pubchem.ncbi.nlm.nih.gov | |
Description | Data deposited in or computed by PubChem | |
Molecular Weight |
970.5 g/mol |
Source
|
Source | PubChem | |
URL | https://pubchem.ncbi.nlm.nih.gov | |
Description | Data deposited in or computed by PubChem | |
Disclaimer and Information on In-Vitro Research Products
Please be aware that all articles and product information presented on BenchChem are intended solely for informational purposes. The products available for purchase on BenchChem are specifically designed for in-vitro studies, which are conducted outside of living organisms. In-vitro studies, derived from the Latin term "in glass," involve experiments performed in controlled laboratory settings using cells or tissues. It is important to note that these products are not categorized as medicines or drugs, and they have not received approval from the FDA for the prevention, treatment, or cure of any medical condition, ailment, or disease. We must emphasize that any form of bodily introduction of these products into humans or animals is strictly prohibited by law. It is essential to adhere to these guidelines to ensure compliance with legal and ethical standards in research and experimentation.