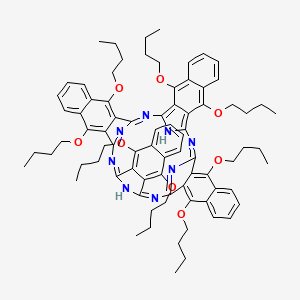
5,9,14,18,23,27,32,36-Octabutoxy-2,3-naphthalocyanine
- Click on QUICK INQUIRY to receive a quote from our team of experts.
- With the quality product at a COMPETITIVE price, you can focus more on your research.
Overview
Description
5,9,14,18,23,27,32,36-Octabutoxy-2,3-naphthalocyanine is an organic compound that belongs to the naphthalocyanine family. It is a derivative of phthalocyanine, which is known for its intense color and stability. This compound is characterized by the presence of eight butoxy groups attached to the naphthalocyanine core, which enhances its solubility and alters its electronic properties. It is commonly used in various applications, including optoelectronics and photonics, due to its unique optical and electronic characteristics .
Mechanism of Action
Target of Action
Similar compounds are known to interact with various cellular structures due to their photonic and optical properties .
Mode of Action
It is known to exhibit strong absorption and fluorescence characteristics in solution , which suggests it may interact with its targets through energy transfer mechanisms.
Result of Action
Its strong absorption and fluorescence characteristics suggest it may have applications in photonic and optical materials .
Action Environment
The compound is stable but gradually decomposes under light exposure . This suggests that environmental factors such as light intensity and exposure duration could influence the compound’s action, efficacy, and stability.
Preparation Methods
Synthetic Routes and Reaction Conditions
The synthesis of 5,9,14,18,23,27,32,36-Octabutoxy-2,3-naphthalocyanine typically involves the reaction of naphthalocyanine with butanol in the presence of a catalyst. The reaction is carried out under reflux conditions to ensure complete substitution of the hydrogen atoms with butoxy groups. The general reaction scheme is as follows:
Starting Materials: Naphthalocyanine and butanol.
Catalyst: Copper(II) chloride or copper(II) acetate.
Reaction Conditions: Reflux in an inert atmosphere (e.g., nitrogen or argon) for several hours.
Purification: The product is purified by column chromatography or recrystallization to obtain the pure compound
Industrial Production Methods
In an industrial setting, the production of this compound follows a similar synthetic route but on a larger scale. The process involves the use of large reactors and continuous flow systems to ensure efficient production. The reaction conditions are optimized to maximize yield and minimize by-products. The final product is subjected to rigorous quality control to ensure its purity and consistency .
Chemical Reactions Analysis
Types of Reactions
5,9,14,18,23,27,32,36-Octabutoxy-2,3-naphthalocyanine undergoes various chemical reactions, including:
Oxidation: The compound can be oxidized to form quinone derivatives.
Reduction: Reduction reactions can lead to the formation of reduced naphthalocyanine species.
Substitution: The butoxy groups can be substituted with other functional groups under appropriate conditions
Common Reagents and Conditions
Oxidation: Common oxidizing agents include potassium permanganate and chromium trioxide.
Reduction: Reducing agents such as sodium borohydride and lithium aluminum hydride are used.
Substitution: Substitution reactions often require the use of strong bases or acids as catalysts
Major Products Formed
Oxidation: Quinone derivatives.
Reduction: Reduced naphthalocyanine species.
Substitution: Various substituted naphthalocyanine derivatives depending on the substituent introduced
Scientific Research Applications
Photothermal Therapy
Overview : Photothermal therapy (PTT) is an innovative approach in cancer treatment that utilizes light-absorbing agents to convert light energy into heat, thereby inducing localized hyperthermia in tumor tissues.
Application of Octabutoxy-2,3-naphthalocyanine :
- Mechanism : The compound acts as a photosensitizer that absorbs near-infrared (NIR) light (with an absorption peak around 867 nm), which is advantageous for deep tissue penetration without damaging surrounding healthy tissues .
- Case Studies : Research indicates that this compound exhibits significant efficacy in PTT by effectively inducing cell death in cancer cell lines when exposed to NIR light. Studies have demonstrated a marked decrease in cell viability post-treatment .
Dyeing and Pigmentation
Overview : The compound is utilized as a dye in various industrial applications due to its vibrant color and chemical stability.
Application in Textiles and Coatings :
- Properties : Its excellent solubility in organic solvents makes it suitable for use in textile dyeing processes and coatings for paints and inks .
- Environmental Considerations : The use of supercritical carbon dioxide as a solvent for dyeing processes has been explored to mitigate environmental impacts associated with traditional dyeing methods. This compound's stability under such conditions enhances its applicability .
Water Purification
Overview : The compound has been investigated for its potential role in water treatment processes.
Mechanism :
- Photodegradation of Contaminants : Due to its photochemical properties, this compound can facilitate the degradation of organic pollutants under light irradiation. This property is particularly useful for the removal of dyes from wastewater .
- Research Findings : Studies have shown that using this compound can lead to significant reductions in chemical oxygen demand (COD) during the purification process .
Nanomaterial Synthesis
Overview : The compound serves as a precursor for synthesizing various nanomaterials with potential applications in electronics and photonics.
Applications :
- Nanoparticle Formation : Research has indicated that this compound can be used to create nanoparticles that exhibit enhanced optical properties beneficial for solar cells and photodetectors .
Summary Table of Applications
Application Area | Description | Key Benefits |
---|---|---|
Photothermal Therapy | Utilizes NIR light to induce hyperthermia in tumors | Effective cancer treatment with minimal side effects |
Dyeing and Pigmentation | Used as a dye in textiles and coatings | Strong color stability and environmental benefits |
Water Purification | Facilitates photodegradation of pollutants | Significant reduction in COD during treatment |
Nanomaterial Synthesis | Serves as a precursor for advanced nanomaterials | Enhanced optical properties for electronic applications |
Comparison with Similar Compounds
Similar Compounds
Copper(II) phthalocyanine: A well-known phthalocyanine derivative with similar optical properties but different solubility characteristics.
Nickel(II) 5,9,14,18,23,27,32,36-Octabutoxy-2,3-naphthalocyanine: Similar structure but with nickel as the central metal ion instead of copper.
Zinc(II) this compound: Similar structure but with zinc as the central metal ion .
Uniqueness
This compound is unique due to its enhanced solubility and altered electronic properties compared to other phthalocyanine derivatives. The presence of eight butoxy groups significantly improves its solubility in organic solvents, making it more versatile for various applications. Additionally, the electronic properties of the compound are modulated by the butoxy groups, leading to unique optical and electronic characteristics .
Biological Activity
5,9,14,18,23,27,32,36-Octabutoxy-2,3-naphthalocyanine (often abbreviated as ONc) is a synthetic compound belonging to the phthalocyanine family. Its unique structure and properties make it a subject of interest in various fields including photothermal therapy and antimicrobial applications. This article provides a comprehensive overview of the biological activity of ONc based on diverse research findings.
- Molecular Formula : C₈₀H₈₈N₈O₈
- Molecular Weight : 1348.30 g/mol
- Dye Content : 95%
- Absorption Maximum (λmax) : 867 nm
Photothermal Therapy (PTT)
ONc has been investigated for its potential in photothermal therapy. PTT utilizes light-absorbing agents to convert light into heat, selectively destroying cancer cells while sparing surrounding healthy tissue. Research has shown that ONc can effectively absorb near-infrared (NIR) light, making it suitable for this application.
Case Study : A study demonstrated that ONc could induce significant thermal effects in cancer cells when exposed to NIR light. The compound's high absorbance at 867 nm allows for deeper tissue penetration compared to visible light, enhancing its therapeutic efficacy .
Antimicrobial Activity
Recent studies have highlighted the antimicrobial properties of ONc against various bacterial strains. The mechanism is believed to involve the generation of reactive oxygen species (ROS) upon light activation.
Research Findings :
- Antibacterial Efficacy : In vitro studies have shown that ONc exhibits significant antibacterial activity against Gram-positive and Gram-negative bacteria. The minimal inhibitory concentration (MIC) values were determined to be low, indicating strong antibacterial potential .
- Mechanism of Action : The antimicrobial effect is attributed to the photodynamic action of ONc when irradiated with light. This process leads to oxidative stress in bacterial cells, resulting in cell death .
Quantum Chemical Calculations
Quantum chemical calculations have been employed to understand the electronic structure and reactivity of ONc. These studies provide insights into its stability and interaction with biological molecules.
- Computational Methods : Density Functional Theory (DFT) calculations have been used to optimize the molecular geometry of ONc and predict its reactivity patterns.
- Findings : The calculations suggest that ONc has favorable energy states for electron transfer processes, which are crucial for its photodynamic activity .
Summary Table of Biological Activities
Properties
IUPAC Name |
5,12,18,25,31,38,44,51-octabutoxy-2,15,28,41,53,54,55,56-octazatridecacyclo[40.10.1.13,14.116,27.129,40.04,13.06,11.017,26.019,24.030,39.032,37.043,52.045,50]hexapentaconta-1,3,5,7,9,11,13,15,17,19,21,23,25,27(55),28,30,32,34,36,38,40,42(53),43,45,47,49,51-heptacosaene |
Source
|
---|---|---|
Source | PubChem | |
URL | https://pubchem.ncbi.nlm.nih.gov | |
Description | Data deposited in or computed by PubChem | |
InChI |
InChI=1S/C80H90N8O8/c1-9-17-41-89-65-49-33-25-26-34-50(49)66(90-42-18-10-2)58-57(65)73-81-74(58)86-76-61-62(70(94-46-22-14-6)54-38-30-29-37-53(54)69(61)93-45-21-13-5)78(83-76)88-80-64-63(71(95-47-23-15-7)55-39-31-32-40-56(55)72(64)96-48-24-16-8)79(84-80)87-77-60-59(75(82-77)85-73)67(91-43-19-11-3)51-35-27-28-36-52(51)68(60)92-44-20-12-4/h25-40H,9-24,41-48H2,1-8H3,(H2,81,82,83,84,85,86,87,88) |
Source
|
Source | PubChem | |
URL | https://pubchem.ncbi.nlm.nih.gov | |
Description | Data deposited in or computed by PubChem | |
InChI Key |
BFXKASLQHBYWIJ-UHFFFAOYSA-N |
Source
|
Source | PubChem | |
URL | https://pubchem.ncbi.nlm.nih.gov | |
Description | Data deposited in or computed by PubChem | |
Canonical SMILES |
CCCCOC1=C2C=CC=CC2=C(C3=C4NC(=C31)N=C5C6=C(C7=CC=CC=C7C(=C6C(=N5)N=C8C9=C(C1=CC=CC=C1C(=C9C(=NC1=NC(=N4)C2=C(C3=CC=CC=C3C(=C21)OCCCC)OCCCC)N8)OCCCC)OCCCC)OCCCC)OCCCC)OCCCC |
Source
|
Source | PubChem | |
URL | https://pubchem.ncbi.nlm.nih.gov | |
Description | Data deposited in or computed by PubChem | |
Molecular Formula |
C80H90N8O8 |
Source
|
Source | PubChem | |
URL | https://pubchem.ncbi.nlm.nih.gov | |
Description | Data deposited in or computed by PubChem | |
Molecular Weight |
1291.6 g/mol |
Source
|
Source | PubChem | |
URL | https://pubchem.ncbi.nlm.nih.gov | |
Description | Data deposited in or computed by PubChem | |
Q1: How does the structure of 5,9,14,18,23,27,32,36-Octabutoxy-2,3-naphthalocyanine contribute to its potential as a material for organic thin-film transistors (OTFTs)?
A2: The presence of the octabutoxy groups in this naphthalocyanine derivative enhances its solubility, facilitating the fabrication of thin films via spin-coating techniques []. Additionally, annealing these films leads to a red-shift in the Q-band, suggesting improved π-orbital overlap within the naphthalocyanine ring system []. This enhanced orbital overlap contributes to the compound's semiconducting properties, making it a suitable candidate for OTFTs [].
Q2: What makes this compound a promising candidate for chemical vapor sensing applications?
A3: OTFT sensors fabricated using this compound demonstrate enhanced sensitivity and selectivity compared to traditional phthalocyanine-based sensors []. Exposure to various analytes induces changes in the OTFTs' electrical properties, such as mobility and threshold voltage, enabling the detection of specific vapors []. This sensing capability is attributed to the compound's ability to interact with analytes through mechanisms like electron donation via hydrogen bonding and stabilization of charge within the semiconductor film [].
Q3: How do different central metal ions influence the photophysical properties of 5,9,14,18,23,27,32,36-Octabutoxy-2,3-naphthalocyanines?
A4: Research suggests that the nature of the central metal ion significantly impacts the excited state dynamics and electronic properties of these compounds []. For example, studies employing transient absorption spectroscopy, combined with DFT/TDDFT calculations, reveal distinct differences in excited state lifetimes and energy transfer pathways depending on the incorporated metal ion []. This highlights the potential for tailoring the photophysical properties of 5,9,14,18,23,27,32,36-Octabutoxy-2,3-naphthalocyanines for specific applications by carefully selecting the central metal ion.
Q4: How does quantum chemistry contribute to understanding the corrosion inhibition properties of this compound and similar compounds?
A5: Quantum chemical calculations offer valuable insights into the interaction of these molecules with metal surfaces, aiding in the rationalization of their corrosion inhibition efficacies []. Parameters such as the energy of the highest occupied molecular orbital (HOMO) and lowest unoccupied molecular orbital (LUMO) can be calculated and correlated with experimental inhibition efficiency data. This allows researchers to establish structure-activity relationships and gain a deeper understanding of the factors governing the performance of these compounds as corrosion inhibitors [].
Disclaimer and Information on In-Vitro Research Products
Please be aware that all articles and product information presented on BenchChem are intended solely for informational purposes. The products available for purchase on BenchChem are specifically designed for in-vitro studies, which are conducted outside of living organisms. In-vitro studies, derived from the Latin term "in glass," involve experiments performed in controlled laboratory settings using cells or tissues. It is important to note that these products are not categorized as medicines or drugs, and they have not received approval from the FDA for the prevention, treatment, or cure of any medical condition, ailment, or disease. We must emphasize that any form of bodily introduction of these products into humans or animals is strictly prohibited by law. It is essential to adhere to these guidelines to ensure compliance with legal and ethical standards in research and experimentation.