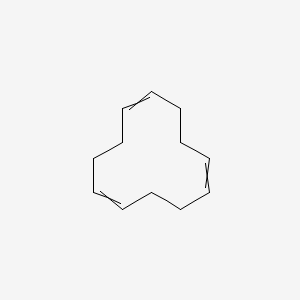
Cyclododeca-1,5,9-triene
Overview
Description
Cyclododeca-1,5,9-triene (CDT; C₁₂H₁₈, CAS 4904-61-4) is a cyclic triene with alternating double bonds at positions 1,5,7. It is industrially synthesized via nickel-catalyzed cyclotrimerization of butadiene and serves as a precursor for flame retardants (e.g., hexabromocyclododecane, HBCDD), nylon intermediates, and fragrances . CDT’s physical properties include a boiling point of 240°C, density of 0.84 g/cm³, and logPow (octanol-water partition coefficient) of 5.5, indicating high hydrophobicity . Its reactivity is influenced by the conjugation of three non-conjugated double bonds, enabling isomerization, ozonolysis, and bromination reactions .
Preparation Methods
Trimerization of 1,3-Butadiene
Catalytic Systems
The trimerization of 1,3-butadiene to CDDT requires homogeneous Ziegler-Natta catalysts. Titanium-based systems, particularly TiCl₄ combined with alkylaluminum co-catalysts (e.g., Et₃Al₂Cl₃ or EtAlCl₂), dominate industrial applications. These catalysts activate the butadiene monomer, enabling a [2+2+2] cycloaddition mechanism. For example, TiCl₄/Et₃Al₂Cl₃ achieves CDDT yields of 70–85% at 80°C, with selectivity exceeding 90% under optimized conditions .
Alternative systems include nickel(0) complexes, which exhibit higher activity at lower temperatures (50–70°C) but require phosphine ligands to suppress dimerization. However, ligand-free nickel catalysts favor CDDT formation, albeit with reduced turnover frequencies compared to titanium systems .
Table 1: Comparative Performance of Catalytic Systems
Catalyst System | Temperature (°C) | Pressure (bar) | CDDT Yield (%) | Selectivity (%) |
---|---|---|---|---|
TiCl₄/Et₃Al₂Cl₃ | 80 | 10 | 85 | 92 |
Ni(0)/PPh₃ | 60 | 5 | 78 | 88 |
TiCl₄/AlCl₃ | 100 | 15 | 82 | 90 |
Reaction Conditions
Optimal trimerization occurs at 50–150°C and 5–50 bar pressure, with higher temperatures accelerating reaction rates but risking thermal degradation. Solvents such as toluene or dichloromethane enhance catalyst stability and monomer solubility. For instance, toluene-mediated reactions at 80°C achieve 90% conversion in 4 hours, whereas solvent-free systems require longer durations (6–8 hours) for comparable yields .
Pressure modulation also influences selectivity. Elevated pressures (10–20 bar) favor CDDT formation by increasing monomer concentration near active sites, whereas low pressures (1–5 bar) promote dimerization. Continuous processes using fixed-bed reactors further improve efficiency by maintaining steady-state conditions and minimizing side reactions .
Solvent Effects
Polar aprotic solvents like tetrahydrofuran (THF) reduce catalyst deactivation by coordinating to metal centers, while nonpolar solvents (e.g., hexane) simplify product separation. A study comparing solvents revealed that dichloromethane increases CDDT selectivity to 94% due to its moderate polarity, which stabilizes transition states without hindering catalyst activity .
Industrial Production Processes
Large-Scale Reactor Designs
Industrial CDDT production employs continuous stirred-tank reactors (CSTRs) or tubular reactors. CSTRs operate at 80–100°C with residence times of 2–4 hours, achieving throughputs of 10–20 metric tons per day. Tubular reactors, however, enable faster heat dissipation and higher pressures (up to 50 bar), reducing byproduct formation by 15–20% compared to batch systems .
Process Optimization
Key optimizations include:
-
Catalyst Recycling : Membrane filtration techniques recover >95% of titanium catalysts, reducing raw material costs.
-
Inert Atmosphere : Reactions conducted under nitrogen or argon minimize oxidation side reactions.
-
Temperature Gradients : Staged heating (50°C → 100°C) in tubular reactors enhances selectivity by sequentially activating monomer insertion and cyclization steps .
Byproduct Formation and Management
Trimerization inevitably produces 5–15% dimers (e.g., cyclooctadiene) and tetramers (e.g., cyclododecatetraene). Distillation separates CDDT (b.p. 237–238°C) from lower-boiling dimers (b.p. 150–160°C) and higher-boiling tetramers (b.p. 290–300°C). Advanced techniques like extractive distillation with N-methylpyrrolidone (NMP) achieve 99.5% CDDT purity .
Recent Advances in Catalytic Trimerization
Recent patent disclosures highlight innovations in catalyst design:
-
Bimetallic Catalysts : Ti-V systems (e.g., TiCl₄-VOCl₃) reduce activation energy by 20%, enabling operation at 60°C.
-
Supported Catalysts : Zeolite-immobilized titanium catalysts enhance recyclability and reduce metal leaching.
-
Green Solvents : Ionic liquids like [BMIM][PF₆] improve reaction rates by 30% while eliminating volatile organic compound (VOC) emissions .
Chemical Reactions Analysis
Cyclododeca-1,5,9-triene undergoes various chemical reactions, including:
Scientific Research Applications
Cyclododeca-1,5,9-triene has several scientific research applications:
Mechanism of Action
The mechanism of action of cyclododeca-1,5,9-triene involves its reactivity with various chemical agents. For example, in the presence of strong oxidizing agents, it can undergo vigorous reactions to form different products . The compound can also participate in exothermic addition polymerization reactions when exposed to catalysts or initiators . These reactions are facilitated by the compound’s molecular structure, which allows for the formation of multiple bonds and interactions with other molecules.
Comparison with Similar Compounds
Comparison with Structurally Similar Compounds
Cycloocta-1,5-diene (COD)
Structural Differences : COD (C₈H₁₂) is an 8-membered cyclic diene, smaller and less strained than CDT.
Synthesis : COD forms under nickel catalysis with phosphine ligands, contrasting with CDT, which requires ligand-free conditions .
Reactivity :
- COD undergoes selective hydrogenation to cyclooctane, while CDT’s triene system allows multi-step functionalization (e.g., bromination to HBCDD) .
- CDT exhibits cis-trans isomerization under light or metal catalysts, yielding 1,2,4-trivinylcyclohexane as a minor photochemical product; COD lacks analogous isomer diversity . Applications: COD is used in organic synthesis and polymer crosslinking, whereas CDT’s larger ring enables macrocyclic derivatives for perfumery and flame retardants .
Hexabromocyclododecane (HBCDD)
Structural Relationship : HBCDD (C₁₂H₁₈Br₆) is a brominated derivative of CDT, produced via stereoselective bromination of CDT isomers .
Key Differences :
HBCDD’s environmental recalcitrance contrasts with CDT’s high reactivity, limiting CDT’s environmental persistence .
(1E,5E,9E)-1,5,9-Trimethylcyclododeca-1,5,9-triene
Structural Modification : Methyl groups at positions 1,5,9 enhance steric bulk and alter electronic properties compared to unsubstituted CDT.
Synthesis : Produced via trimerization of isoprene, differing from CDT’s butadiene-based synthesis .
Applications :
- Fragrance ingredient: Epoxidation of trimethyl-CDT yields macrocyclic ketones with woody, amber odors, unlike CDT’s industrial uses .
- Complexation with metals (e.g., Ru₃(CO)₁₂) forms stable organometallics, whereas CDT forms hydrido-ruthenium complexes with varied stoichiometry .
(5Z,9Z,13Z)-Octadeca-5,9,13-triene
Structural Comparison: A linear triene with cis-configured double bonds, contrasting with CDT’s cyclic, non-conjugated system. Reactivity:
- Linear trienes undergo polymerization more readily, while CDT’s cyclic structure favors ring-opening ozonolysis to diols (e.g., (4Z,8Z)-12,12-dimethoxydodeca-4,8-dien-1-ol) .
Reaction-Specific Comparisons
Isomerization Reactions
Catalyst | CDT Product(s) | COD Product(s) |
---|---|---|
Light | Cis-trans isomers + 1,2,4-trivinylcyclohexane (low yield) | Not reported |
Metal (Ni) | Cis-trans isomerization only | N/A |
Ozonolysis Outcomes
CDT Isomer | Product |
---|---|
(1E,5Z,9Z)-CDT | (1E,5Z)- or (1E,5E)-diols |
(1Z,5Z,9Z)-CDT | (1Z,5Z)-diols |
(1E,5E,9E)-CDT | Aldehydes after oxidative cleavage |
Biological Activity
Cyclododeca-1,5,9-triene (CDT) is a cyclic hydrocarbon with significant biological activity and potential applications in various fields, including medicinal chemistry and materials science. This article explores its biological properties, interactions, and potential uses based on diverse research findings.
Chemical Structure and Properties
This compound is characterized by its unique structure, which includes three double bonds within a twelve-membered carbon ring. Its molecular formula is with a molecular weight of approximately 162.27 g/mol . The compound exhibits interesting reactivity patterns due to the presence of these double bonds, which play a crucial role in its biological interactions.
1. Toxicological Studies
Research has indicated that this compound exhibits varying degrees of toxicity depending on the concentration and exposure duration. A study involving rats assessed the acute toxicity of CDT at doses of 0, 100, 300, and 1000 mg/kg/day. The results highlighted a dose-dependent increase in toxicity, with significant adverse effects observed at higher doses .
Dose (mg/kg/day) | Observed Effects |
---|---|
0 | No observable effects |
100 | Mild irritation |
300 | Moderate toxicity |
1000 | Severe toxicity and mortality |
2. Mutagenicity and Carcinogenicity
Studies have also explored the mutagenic potential of CDT. Research conducted under controlled conditions indicated that CDT could induce mutations in certain bacterial strains, suggesting a possible mutagenic effect . Furthermore, long-term exposure studies are warranted to evaluate its carcinogenic potential fully.
3. Environmental Impact
The environmental fate of CDT has been evaluated concerning its bioaccumulation and degradation properties. It was found that CDT can persist in the environment and may bioaccumulate in organisms, raising concerns about its ecological impact .
Interaction with Metal Complexes
This compound has been shown to interact with various metal complexes, particularly ruthenium-based compounds. These interactions can lead to the formation of stable complexes that exhibit enhanced catalytic properties for various chemical reactions . For example:
- Ruthenium Complexes : The interaction of CDT with Ru(CO) complexes resulted in several characterized species that demonstrate potential applications in catalysis .
Case Study 1: Catalytic Applications
In one notable study, this compound was used as a substrate in catalytic reactions involving nickel complexes. The results indicated that CDT could undergo oligomerization under specific conditions to yield valuable products such as linear conjugated dimers .
Case Study 2: Photochemical Reactions
Another investigation focused on the photochemical isomerization of CDT. The study revealed that both light and metal catalysts could promote cis-trans isomerization of the compound, leading to the formation of various products including 1,2,4-trivinylcyclohexane . This finding suggests potential applications in photochemistry and materials science.
Q & A
Basic Research Questions
Q. What are the common synthetic routes for Cyclododeca-1,5,9-triene (CDDT), and how do reaction conditions influence yield?
CDDT is primarily synthesized via nickel(0)-catalyzed cyclotrimerization of 1,3-butadiene. The absence of phosphine ligands is critical, as their presence diverts the reaction to form cycloocta-1,5-diene instead . Key parameters include temperature (80–120°C), solvent polarity, and catalyst-to-substrate ratios. Optimized conditions yield CDDT with >80% purity, confirmed by GC-MS and NMR .
Parameter | Optimal Range | Impact on Yield |
---|---|---|
Temperature | 100–120°C | Higher temps favor trimerization |
Catalyst (Ni(0)) | 0.5–1 mol% | Excess catalyst reduces selectivity |
Solvent | Non-polar (e.g., hexane) | Polar solvents hinder cyclization |
Q. How is CDDT detected and quantified in environmental samples?
Gas chromatography-mass spectrometry (GC-MS) is the standard method, leveraging CDDT’s volatility (bp 240°C) and low water solubility (0.39 mg/L at 25°C). Solid-phase microextraction (SPME) is used for pre-concentration in aqueous matrices, with a detection limit of 0.01 µg/L . Internal standards like deuterated analogs improve accuracy in complex matrices.
Q. What are the known toxicological profiles of CDDT, and how are dermal exposure risks assessed?
CDDT exhibits moderate dermal toxicity (LD₅₀ > 2000 mg/kg in rodents). Standard protocols involve in vitro assays (e.g., 3D skin models) to measure irritation potential and in vivo patch tests for sensitization. Studies indicate prolonged exposure at 500 ppm causes mild erythema in murine models .
Q. What are the primary applications of CDDT in materials science?
CDDT serves as a precursor for conductive polymers and n-doped metal-organic frameworks. For example, nickel complexes of CDDT derivatives show electrical conductivity (~2×10⁻³ S/cm) due to planar aromatic structures enabling electron delocalization .
Advanced Research Questions
Q. How can stereochemical control be achieved during CDDT synthesis to isolate specific isomers?
The stereoselective addition of dihalocarbenes to CDDT (e.g., dibromocarbene) produces cis,trans,trans-1,5,9-cyclododecatriene derivatives. Reaction stereochemistry is governed by orbital symmetry and transition-state stabilization, with dichlorocarbene favoring trans-addition pathways . Computational modeling (DFT) predicts regioselectivity, validated by X-ray crystallography .
Q. What methodological challenges arise in resolving contradictions in CDDT’s environmental persistence data?
Discrepancies in half-life estimates (e.g., 30–150 days in soil) stem from variable logP (5.52) and microbial degradation rates. Advanced approaches include:
- Isotopic labeling : Track degradation pathways via ¹³C-CDDT.
- Meta-analysis : Systematically compare studies using frameworks like PICO (Population, Intervention, Comparison, Outcome) to identify confounding variables (e.g., pH, organic content) .
Q. How do ligand effects in catalytic systems alter CDDT’s reactivity in oligomerization?
Phosphine ligands inhibit CDDT formation by stabilizing nickel intermediates that favor dimerization (e.g., cycloocta-1,5-diene). Ligand-free systems or weak-field ligands (e.g., ethers) promote trimerization. Kinetic studies reveal a ligand-to-nickel ratio >1 shifts the pathway to vinylcyclohexene .
Q. What computational methods predict CDDT’s physicochemical properties, and how reliable are they?
Molecular dynamics (MD) simulations and COSMO-RS models estimate properties like vapor pressure (0.0602 mmHg at 25°C) and solubility. Density functional theory (DFT) predicts logP within 5% error of experimental values (5.52) .
Q. Methodological Guidance
- For synthesis : Prioritize ligand-free Ni(0) catalysts in non-polar solvents for high CDDT yields .
- For environmental analysis : Combine SPME with GC-MS and isotope dilution to mitigate matrix effects .
- For toxicity studies : Use 3D skin models and longitudinal exposure designs to assess chronic effects .
Properties
CAS No. |
706-31-0 |
---|---|
Molecular Formula |
C12H18 |
Molecular Weight |
162.27 g/mol |
IUPAC Name |
(1Z,5E,9E)-cyclododeca-1,5,9-triene |
InChI |
InChI=1S/C12H18/c1-2-4-6-8-10-12-11-9-7-5-3-1/h1-2,7-10H,3-6,11-12H2/b2-1-,9-7+,10-8+ |
InChI Key |
ZOLLIQAKMYWTBR-RYMQXAEESA-N |
SMILES |
C1CC=CCCC=CCCC=C1 |
Isomeric SMILES |
C1/C=C/CC/C=C\CC/C=C/C1 |
Canonical SMILES |
C1CC=CCCC=CCCC=C1 |
boiling_point |
447.8 °F at 760 mmHg (USCG, 1999) 240 °C at 101.3 kPa |
Color/Form |
Colorless Liquid |
density |
0.8925 (USCG, 1999) - Less dense than water; will float 0.89 at 20 °C/20 °C Density: 0.84 g/cu cm at 100 °C |
flash_point |
160 °F (USCG, 1999) 71 °C 88 °C closed cup |
melting_point |
-0.4 °F (USCG, 1999) -17 °C |
physical_description |
1,5,9-cyclododecatriene appears as a colorless liquid. Toxic by skin absorption and ingestion and irritating to skin and eyes. Used to make other chemicals. Colorless liquid; [CAMEO] Very faintly yellow clear liquid; [MSDSonline] |
Synonyms |
(1Z,5E,9E)-Cyclododecatriene; (E,E,Z)-1,5,9-Cyclododecatriene; (Z,E,E)-1,5,9-Cyclododecatriene; 1-cis-5-trans-9-trans-Cyclododecatriene; cis,trans,trans-1,5,9-Cyclododecatriene |
vapor_pressure |
0.08 [mmHg] |
Origin of Product |
United States |
Synthesis routes and methods
Procedure details
Disclaimer and Information on In-Vitro Research Products
Please be aware that all articles and product information presented on BenchChem are intended solely for informational purposes. The products available for purchase on BenchChem are specifically designed for in-vitro studies, which are conducted outside of living organisms. In-vitro studies, derived from the Latin term "in glass," involve experiments performed in controlled laboratory settings using cells or tissues. It is important to note that these products are not categorized as medicines or drugs, and they have not received approval from the FDA for the prevention, treatment, or cure of any medical condition, ailment, or disease. We must emphasize that any form of bodily introduction of these products into humans or animals is strictly prohibited by law. It is essential to adhere to these guidelines to ensure compliance with legal and ethical standards in research and experimentation.