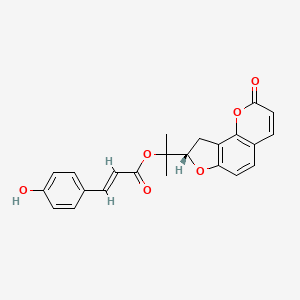
(+)-Angelmarin
- Click on QUICK INQUIRY to receive a quote from our team of experts.
- With the quality product at a COMPETITIVE price, you can focus more on your research.
Overview
Description
(+)-Angelmarin is a naturally occurring sesquiterpene lactone isolated from the plant Angelica pubescens. It is known for its diverse biological activities, including anti-inflammatory, anti-cancer, and anti-microbial properties. The compound has a unique structure characterized by a lactone ring fused to a sesquiterpene backbone, which contributes to its biological activity.
Scientific Research Applications
(+)-Angelmarin has a wide range of scientific research applications:
Chemistry: It is used as a starting material for the synthesis of various bioactive compounds.
Biology: The compound is studied for its effects on cellular processes, including apoptosis and cell cycle regulation.
Medicine: this compound is investigated for its potential therapeutic applications in treating inflammatory diseases, cancer, and microbial infections.
Industry: The compound is used in the development of natural product-based pharmaceuticals and as a lead compound in drug discovery.
Preparation Methods
Synthetic Routes and Reaction Conditions: The synthesis of (+)-Angelmarin involves several steps, starting from commercially available precursors. The key steps include the formation of the sesquiterpene backbone, followed by the introduction of the lactone ring. The reaction conditions typically involve the use of strong acids or bases, high temperatures, and specific catalysts to facilitate the formation of the desired product.
Industrial Production Methods: Industrial production of this compound is often achieved through the extraction from Angelica pubescens. The plant material is subjected to solvent extraction, followed by purification using chromatographic techniques. This method ensures the isolation of high-purity this compound suitable for various applications.
Chemical Reactions Analysis
Types of Reactions: (+)-Angelmarin undergoes several types of chemical reactions, including:
Oxidation: The compound can be oxidized to form various derivatives, which may exhibit different biological activities.
Reduction: Reduction reactions can modify the lactone ring, leading to the formation of different analogs.
Substitution: Substitution reactions can introduce different functional groups into the molecule, altering its properties.
Common Reagents and Conditions:
Oxidation: Common oxidizing agents include potassium permanganate and chromium trioxide.
Reduction: Reducing agents such as lithium aluminum hydride and sodium borohydride are often used.
Substitution: Various reagents, including halogens and alkylating agents, are used under controlled conditions to achieve the desired substitutions.
Major Products Formed: The major products formed from these reactions include oxidized derivatives, reduced analogs, and substituted compounds, each with potentially unique biological activities.
Mechanism of Action
The mechanism of action of (+)-Angelmarin involves its interaction with specific molecular targets and pathways:
Molecular Targets: The compound targets various enzymes and receptors involved in inflammation, cell proliferation, and apoptosis.
Pathways Involved: this compound modulates signaling pathways such as the nuclear factor-kappa B (NF-κB) pathway, which plays a crucial role in inflammation and cancer.
Comparison with Similar Compounds
Parthenolide: Another sesquiterpene lactone with anti-inflammatory and anti-cancer properties.
Artemisinin: A sesquiterpene lactone known for its anti-malarial activity.
Costunolide: A sesquiterpene lactone with anti-cancer and anti-inflammatory effects.
Comparison: (+)-Angelmarin is unique due to its specific structure and the presence of a lactone ring fused to a sesquiterpene backbone. This structural uniqueness contributes to its distinct biological activities compared to other similar compounds. While parthenolide and costunolide share some anti-inflammatory and anti-cancer properties, this compound’s specific molecular interactions and pathways provide it with unique therapeutic potential.
Properties
IUPAC Name |
2-[(8S)-2-oxo-8,9-dihydrofuro[2,3-h]chromen-8-yl]propan-2-yl (E)-3-(4-hydroxyphenyl)prop-2-enoate |
Source
|
---|---|---|
Source | PubChem | |
URL | https://pubchem.ncbi.nlm.nih.gov | |
Description | Data deposited in or computed by PubChem | |
InChI |
InChI=1S/C23H20O6/c1-23(2,29-21(26)11-5-14-3-8-16(24)9-4-14)19-13-17-18(27-19)10-6-15-7-12-20(25)28-22(15)17/h3-12,19,24H,13H2,1-2H3/b11-5+/t19-/m0/s1 |
Source
|
Source | PubChem | |
URL | https://pubchem.ncbi.nlm.nih.gov | |
Description | Data deposited in or computed by PubChem | |
InChI Key |
YPULLITUOWRRPG-RXERPNITSA-N |
Source
|
Source | PubChem | |
URL | https://pubchem.ncbi.nlm.nih.gov | |
Description | Data deposited in or computed by PubChem | |
Canonical SMILES |
CC(C)(C1CC2=C(O1)C=CC3=C2OC(=O)C=C3)OC(=O)C=CC4=CC=C(C=C4)O |
Source
|
Source | PubChem | |
URL | https://pubchem.ncbi.nlm.nih.gov | |
Description | Data deposited in or computed by PubChem | |
Isomeric SMILES |
CC(C)([C@@H]1CC2=C(O1)C=CC3=C2OC(=O)C=C3)OC(=O)/C=C/C4=CC=C(C=C4)O |
Source
|
Source | PubChem | |
URL | https://pubchem.ncbi.nlm.nih.gov | |
Description | Data deposited in or computed by PubChem | |
Molecular Formula |
C23H20O6 |
Source
|
Source | PubChem | |
URL | https://pubchem.ncbi.nlm.nih.gov | |
Description | Data deposited in or computed by PubChem | |
Molecular Weight |
392.4 g/mol |
Source
|
Source | PubChem | |
URL | https://pubchem.ncbi.nlm.nih.gov | |
Description | Data deposited in or computed by PubChem | |
Q1: What makes (+)-Angelmarin a promising anticancer agent?
A1: this compound exhibits potent antiausterity activity [, ]. This means it selectively targets cancer cells under nutrient-deprived conditions, a characteristic often observed in resistant tumors. This selectivity is crucial as it could potentially minimize side effects on healthy cells, which are typically in nutrient-rich environments.
Q2: What is known about the structure-activity relationship (SAR) of this compound?
A2: Research suggests that the isoprenyl group in this compound plays a vital role in its anticancer activity [, ]. Modifications to this group have been explored to understand its impact on potency and selectivity. For instance, replacing the isoprenyl group with other substituents led to a decrease in cytotoxicity against pancreatic cancer cell lines, highlighting the importance of this specific structural feature [].
Q3: Which cancer types have been studied in relation to this compound's activity?
A3: A significant portion of the research focuses on this compound's efficacy against pancreatic cancer cells, particularly the PANC-1 cell line [, , , ]. This cell line is known for its aggressive nature and resistance to conventional therapies, making the selective cytotoxicity of this compound particularly relevant.
Q4: What is the mechanism of action of this compound in pancreatic cancer cells?
A4: While the exact mechanism is still under investigation, studies suggest that this compound might induce autophagy in pancreatic cancer cells []. This process, involving the degradation and recycling of cellular components, can lead to cell death under certain circumstances. Research has shown increased conversion of LC3-I to LC3-II, a hallmark of autophagy, upon treatment with this compound [].
Q5: Have any total syntheses of this compound been reported?
A5: Yes, several total syntheses have been developed for this compound, showcasing its importance as a target for chemical synthesis [, , ]. These synthetic routes utilize various strategies and reactions, such as olefin cross-metathesis, Shi epoxidation-cyclization, and copper cyanide-mediated esterification, to efficiently construct the molecule [, ].
Q6: What analytical techniques are commonly employed to study this compound?
A6: Researchers use a variety of techniques, including spectroscopic methods like NMR and mass spectrometry, to characterize and quantify this compound [, ]. In biological studies, cell viability assays, like the MTT assay, are commonly used to assess its antiproliferative activity against cancer cell lines [, ].
Disclaimer and Information on In-Vitro Research Products
Please be aware that all articles and product information presented on BenchChem are intended solely for informational purposes. The products available for purchase on BenchChem are specifically designed for in-vitro studies, which are conducted outside of living organisms. In-vitro studies, derived from the Latin term "in glass," involve experiments performed in controlled laboratory settings using cells or tissues. It is important to note that these products are not categorized as medicines or drugs, and they have not received approval from the FDA for the prevention, treatment, or cure of any medical condition, ailment, or disease. We must emphasize that any form of bodily introduction of these products into humans or animals is strictly prohibited by law. It is essential to adhere to these guidelines to ensure compliance with legal and ethical standards in research and experimentation.