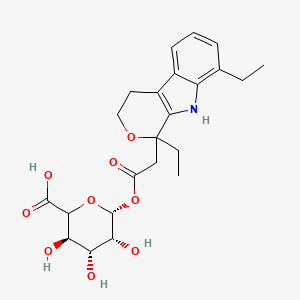
Etodolac Acyl-beta-D-glucuronide
Overview
Description
Etodolac Acyl Glucuronide is a metabolite of etodolac, a non-steroidal anti-inflammatory drug (NSAID) used to treat conditions such as osteoarthritis and rheumatoid arthritis . This compound is formed through the conjugation of etodolac with glucuronic acid, a process facilitated by the enzyme UDP-glucuronosyltransferase . The formation of acyl glucuronides is a common metabolic pathway for carboxylic acid-containing drugs .
Scientific Research Applications
Etodolac Acyl Glucuronide has several scientific research applications, including:
Toxicology Studies: The compound is used to study the potential hepatotoxicity of acyl glucuronides, as these metabolites can form protein adducts that may lead to liver damage.
Drug Metabolism Research: Researchers use Etodolac Acyl Glucuronide to study the metabolic pathways of NSAIDs and the role of glucuronidation in drug clearance.
Pharmacokinetics: The compound is used to investigate the pharmacokinetic properties of etodolac, including its absorption, distribution, metabolism, and excretion.
Mechanism of Action
Target of Action
Etodolac Acyl-beta-D-glucuronide, a metabolite of Etodolac , primarily targets cyclooxygenases (COXs) and lipoxygenases (LOXs) . These enzymes play a crucial role in the inflammatory response .
Mode of Action
The compound’s interaction with its targets results in the inhibition of COXs . This inhibition decreases the synthesis of peripheral prostaglandins involved in mediating inflammation . The anti-inflammatory effects of this compound are due to this ability to inhibit prostaglandin synthesis .
Biochemical Pathways
this compound is formed via glucuronidation of etodolac by the UDP-glucuronosyltransferase (UGT) isoforms UGT1A9, UGT1A10, and UGT2B7 . This process affects the cyclooxygenase pathway, leading to decreased formation of prostaglandin precursors .
Pharmacokinetics
The compound’s ADME (Absorption, Distribution, Metabolism, and Excretion) properties are as follows: this compound is a phase II metabolite of etodolac . It is formed through the conjugation of α-D-glucuronic acid with the carboxylic acid group of etodolac . The rate of degradation of this compound via intramolecular transacylation and hydrolysis has been associated with the degree of protein adduct formation .
Result of Action
The molecular and cellular effects of this compound’s action are primarily related to its anti-inflammatory properties . By inhibiting COXs, it reduces the synthesis of prostaglandins, which are key mediators of inflammation . This leads to relief of signs and symptoms of conditions like rheumatoid arthritis and osteoarthritis .
Action Environment
The action, efficacy, and stability of this compound can be influenced by various environmental factors. For instance, the rate of its degradation via intramolecular transacylation and hydrolysis can impact the degree of protein adduct formation .
Safety and Hazards
Biochemical Analysis
Biochemical Properties
Etodolac Acyl-beta-D-glucuronide plays a role in biochemical reactions as a metabolite of Etodolac . It is formed in the liver and is extensively metabolized . The therapeutic effects of Etodolac, and by extension this compound, are due to its ability to inhibit prostaglandin synthesis .
Cellular Effects
This compound, as a metabolite of Etodolac, may influence cell function by inhibiting the synthesis of prostaglandins involved in fever, pain, swelling, and inflammation
Molecular Mechanism
The molecular mechanism of this compound is closely tied to that of its parent compound, Etodolac. Etodolac binds to the upper portion of the COX enzyme active site and prevents its substrate, arachidonic acid, from entering the active site . It is now known to be more selective for COX-2 than COX-1 .
Temporal Effects in Laboratory Settings
It is known that Etodolac is well absorbed, with maximal plasma concentrations attained within 1 to 2 hours in healthy volunteers .
Dosage Effects in Animal Models
For Etodolac, the initial dose is usually 300 mg orally 2 to 3 times a day or 400 mg to 500 mg orally twice a day .
Metabolic Pathways
This compound is involved in the metabolic pathways of Etodolac. Etodolac is extensively metabolized in the liver, and renal elimination of Etodolac and its metabolites is the primary route of excretion .
Transport and Distribution
It is known that Etodolac is highly plasma protein bound, and undergoes virtually complete biotransformation to oxidised metabolites and acyl-glucuronides .
Preparation Methods
Synthetic Routes and Reaction Conditions: Etodolac Acyl Glucuronide is synthesized through the conjugation of etodolac with glucuronic acid. This reaction is catalyzed by the enzyme UDP-glucuronosyltransferase, which is found in the liver . The reaction typically occurs under physiological conditions, with the enzyme facilitating the transfer of the glucuronic acid moiety to the carboxylic acid group of etodolac .
Industrial Production Methods: Industrial production of Etodolac Acyl Glucuronide involves the use of recombinant UDP-glucuronosyltransferase enzymes to catalyze the conjugation reaction. This process is carried out in bioreactors under controlled conditions to ensure high yield and purity of the product .
Chemical Reactions Analysis
Types of Reactions: Etodolac Acyl Glucuronide undergoes several types of chemical reactions, including:
Transacylation: This reaction involves the transfer of the acyl group from the glucuronide to another molecule, which can lead to the formation of protein adducts.
Common Reagents and Conditions:
Hydrolysis: Typically occurs under acidic or basic conditions, with water acting as the nucleophile.
Transacylation: Can occur under physiological conditions, with nucleophiles such as amino acids or proteins acting as the acceptors.
Major Products Formed:
Hydrolysis: Etodolac and glucuronic acid.
Transacylation: Protein adducts and other acylated molecules.
Comparison with Similar Compounds
- Ibuprofen Acyl Glucuronide
- Naproxen Acyl Glucuronide
- Diclofenac Acyl Glucuronide
Comparison: Etodolac Acyl Glucuronide is unique in its specific formation from etodolac, which has a distinct chemical structure compared to other NSAIDs . While all acyl glucuronides share the potential for protein adduct formation and associated toxicity, the specific reactivity and metabolic pathways can vary based on the parent drug’s structure .
Properties
{ "Design of the Synthesis Pathway": "The synthesis pathway of Etodolac Acyl-beta-D-glucuronide involves the acylation of Etodolac with glucuronic acid to form the glucuronide conjugate.", "Starting Materials": [ "Etodolac", "Glucuronic acid", "Dicyclohexylcarbodiimide (DCC)", "Dimethylaminopyridine (DMAP)", "N,N-Dimethylformamide (DMF)", "Methanol", "Diethyl ether", "Sodium bicarbonate" ], "Reaction": [ "Etodolac is dissolved in DMF and DCC is added to activate the carboxylic acid group.", "Glucuronic acid is dissolved in DMF and DMAP is added as a catalyst.", "The activated Etodolac is added dropwise to the glucuronic acid solution and the reaction mixture is stirred at room temperature for several hours.", "The reaction mixture is then poured into a mixture of methanol and diethyl ether to precipitate the product.", "The product is filtered and washed with diethyl ether to remove any impurities.", "The crude product is dissolved in a solution of sodium bicarbonate and methanol to form the Etodolac Acyl-beta-D-glucuronide salt.", "The salt is filtered and dried to obtain the pure compound." ] } | |
CAS No. |
79541-43-8 |
Molecular Formula |
C23H29NO9 |
Molecular Weight |
463.5 g/mol |
IUPAC Name |
(2S,3S,4S,5R,6S)-6-[2-(1,8-diethyl-4,9-dihydro-3H-pyrano[3,4-b]indol-1-yl)acetyl]oxy-3,4,5-trihydroxyoxane-2-carboxylic acid |
InChI |
InChI=1S/C23H29NO9/c1-3-11-6-5-7-12-13-8-9-31-23(4-2,20(13)24-15(11)12)10-14(25)32-22-18(28)16(26)17(27)19(33-22)21(29)30/h5-7,16-19,22,24,26-28H,3-4,8-10H2,1-2H3,(H,29,30)/t16-,17-,18+,19-,22+,23?/m0/s1 |
InChI Key |
XJZNMEMKZBFUIZ-GWDFKZQESA-N |
Isomeric SMILES |
CCC1=C2C(=CC=C1)C3=C(N2)C(OCC3)(CC)CC(=O)O[C@H]4[C@@H]([C@H]([C@@H]([C@H](O4)C(=O)O)O)O)O |
SMILES |
CCC1=C2C(=CC=C1)C3=C(N2)C(OCC3)(CC)CC(=O)OC4C(C(C(C(O4)C(=O)O)O)O)O |
Canonical SMILES |
CCC1=C2C(=CC=C1)C3=C(N2)C(OCC3)(CC)CC(=O)OC4C(C(C(C(O4)C(=O)O)O)O)O |
Synonyms |
1-(1,8-Diethyl-1,3,4,9-tetrahydropyrano[3,4-b]indole-1-acetate) β-D-Glucopyranuronic Acid; Etodolac Glucuronide; |
Origin of Product |
United States |
Retrosynthesis Analysis
AI-Powered Synthesis Planning: Our tool employs the Template_relevance Pistachio, Template_relevance Bkms_metabolic, Template_relevance Pistachio_ringbreaker, Template_relevance Reaxys, Template_relevance Reaxys_biocatalysis model, leveraging a vast database of chemical reactions to predict feasible synthetic routes.
One-Step Synthesis Focus: Specifically designed for one-step synthesis, it provides concise and direct routes for your target compounds, streamlining the synthesis process.
Accurate Predictions: Utilizing the extensive PISTACHIO, BKMS_METABOLIC, PISTACHIO_RINGBREAKER, REAXYS, REAXYS_BIOCATALYSIS database, our tool offers high-accuracy predictions, reflecting the latest in chemical research and data.
Strategy Settings
Precursor scoring | Relevance Heuristic |
---|---|
Min. plausibility | 0.01 |
Model | Template_relevance |
Template Set | Pistachio/Bkms_metabolic/Pistachio_ringbreaker/Reaxys/Reaxys_biocatalysis |
Top-N result to add to graph | 6 |
Feasible Synthetic Routes
Disclaimer and Information on In-Vitro Research Products
Please be aware that all articles and product information presented on BenchChem are intended solely for informational purposes. The products available for purchase on BenchChem are specifically designed for in-vitro studies, which are conducted outside of living organisms. In-vitro studies, derived from the Latin term "in glass," involve experiments performed in controlled laboratory settings using cells or tissues. It is important to note that these products are not categorized as medicines or drugs, and they have not received approval from the FDA for the prevention, treatment, or cure of any medical condition, ailment, or disease. We must emphasize that any form of bodily introduction of these products into humans or animals is strictly prohibited by law. It is essential to adhere to these guidelines to ensure compliance with legal and ethical standards in research and experimentation.