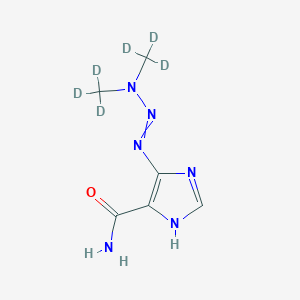
Dacarbazine-d6
- Click on QUICK INQUIRY to receive a quote from our team of experts.
- With the quality product at a COMPETITIVE price, you can focus more on your research.
Overview
Description
Dacarbazine-d6 is intended for use as an internal standard for the quantification of dacarbazine by GC- or LC-MS. Dacarbazine is a DNA alkylating prodrug that is activated by P450 enzymes in liver microsomes. Following activation, it is converted, through a series of reactions, into a methyldiazonium cation that alkylates DNA at all phases of the cell cycle and induces apoptosis. In vitro, dacarbazine inhibits the growth of B16/F1, A-875, and SK-MEL-5 melanoma and non-cancerous WI-38 lung fibroblast and L-02 hepatocyte cell lines (IC50s = 260, 287, 380, 526, and 367 μM, respectively). Dacarbazine toxicity to 518A2 and SK-MEL-28 melanoma cell lines increases in a time-dependent manner with IC50 values of 121 and >400 μM, respectively, following a 1 hour incubation and 2.5 and 50 μM, respectively, following a 96 hour incubation. In vivo, dacarbazine (70 mg/kg, once every 2 days) decreases tumor volume by 59.1% in a B16/F1 murine melanoma model in mice. Formulations containing dacarbazine have been used in the treatment of metastatic melanoma and for Hodgkin's lymphoma in combination with other antineoplastic agents.
Mechanism of Action
Target of Action
Dacarbazine-d6, also known as Dacarbazine, is an antineoplastic agent primarily used to treat malignant melanoma and Hodgkin’s disease . It is an alkylating agent that interferes with DNA synthesis . The primary targets of Dacarbazine are the DNA of cancer cells .
Mode of Action
It appears to exert cytotoxic effects via its action as an alkylating agent . Other theories include DNA synthesis inhibition by its action as a purine analog, and interaction with SH groups .
Biochemical Pathways
Dacarbazine is metabolized in the liver by cytochromes P450 to form the reactive N-demethylated species 5-[3-hydroxymethyl-3-methyl-triazen-1-yl]-imidazole-4-carboxamide (HMMTIC) and 5-[3-methyl-triazen-1-yl]-imidazole-4-carboxamide (MTIC) . The DTIC N-demethylation involved in MTIC formation by human liver microsomes is catalyzed by CYP1A1, CYP1A2, and CYP2E1 .
Pharmacokinetics
After intravenous administration of Dacarbazine, the volume of distribution exceeds total body water content suggesting localization in some body tissue, probably the liver . The drug is extensively metabolized, and its elimination half-life is approximately 5 hours . About 40% of the drug is excreted unchanged in the urine .
Result of Action
Dacarbazine’s action results in disruption of DNA function, cell cycle arrest, and apoptosis . It has been associated with tumor reduction in approximately 30% of patients . The DNA methylation produced by Dacarbazine is dependent upon oxidative N-demethylation by P450s .
Action Environment
The efficacy of Dacarbazine can be influenced by environmental factors such as temperature . For instance, hyperthermia has been shown to potentiate the effect of Dacarbazine, leading to increased cytotoxicity . The stability of dacarbazine can be compromised by light, which causes it to convert into 2-azahypoxanthine, reducing its activity .
Biochemical Analysis
Biochemical Properties
Dacarbazine-d6 interacts with various enzymes and proteins in the body. It is activated by P450 enzymes in liver microsomes . Following activation, it is converted into a methyldiazonium cation that alkylates DNA at all phases of the cell cycle and induces apoptosis .
Cellular Effects
This compound has significant effects on various types of cells and cellular processes. It inhibits the growth of B16/F1, A-875, and SK-MEL-5 melanoma and non-cancerous WI-38 lung fibroblast and L-02 hepatocyte cell lines . It also influences cell function by impacting cell signaling pathways, gene expression, and cellular metabolism .
Molecular Mechanism
The molecular mechanism of this compound involves its action as an alkylating agent . It exerts its effects
Properties
IUPAC Name |
4-[[bis(trideuteriomethyl)amino]diazenyl]-1H-imidazole-5-carboxamide |
Source
|
---|---|---|
Source | PubChem | |
URL | https://pubchem.ncbi.nlm.nih.gov | |
Description | Data deposited in or computed by PubChem | |
InChI |
InChI=1S/C6H10N6O/c1-12(2)11-10-6-4(5(7)13)8-3-9-6/h3H,1-2H3,(H2,7,13)(H,8,9)/i1D3,2D3 |
Source
|
Source | PubChem | |
URL | https://pubchem.ncbi.nlm.nih.gov | |
Description | Data deposited in or computed by PubChem | |
InChI Key |
FDKXTQMXEQVLRF-WFGJKAKNSA-N |
Source
|
Source | PubChem | |
URL | https://pubchem.ncbi.nlm.nih.gov | |
Description | Data deposited in or computed by PubChem | |
Canonical SMILES |
CN(C)N=NC1=C(NC=N1)C(=O)N |
Source
|
Source | PubChem | |
URL | https://pubchem.ncbi.nlm.nih.gov | |
Description | Data deposited in or computed by PubChem | |
Isomeric SMILES |
[2H]C([2H])([2H])N(C([2H])([2H])[2H])N=NC1=C(NC=N1)C(=O)N |
Source
|
Source | PubChem | |
URL | https://pubchem.ncbi.nlm.nih.gov | |
Description | Data deposited in or computed by PubChem | |
Molecular Formula |
C6H10N6O |
Source
|
Source | PubChem | |
URL | https://pubchem.ncbi.nlm.nih.gov | |
Description | Data deposited in or computed by PubChem | |
Molecular Weight |
188.22 g/mol |
Source
|
Source | PubChem | |
URL | https://pubchem.ncbi.nlm.nih.gov | |
Description | Data deposited in or computed by PubChem | |
Q1: What is the role of Dacarbazine-d6 in the research on dacarbazine levels in melanoma patients?
A1: this compound serves as an internal standard (IS) in the liquid chromatography-tandem mass spectrometry (LC-MS/MS) method developed by the researchers []. It's crucial for accurate quantification of dacarbazine in plasma samples.
Q2: How does using this compound as an internal standard improve the accuracy of the LC-MS/MS method for quantifying dacarbazine?
A2: Using this compound, a deuterated form of dacarbazine, compensates for potential variations during sample preparation and analysis. Since it has a nearly identical chemical structure to dacarbazine, it behaves similarly during extraction and ionization but can be differentiated by the mass spectrometer due to its mass difference. By comparing the signal intensity of dacarbazine to the known concentration of this compound, the researchers can accurately determine dacarbazine levels in the plasma samples [].
Disclaimer and Information on In-Vitro Research Products
Please be aware that all articles and product information presented on BenchChem are intended solely for informational purposes. The products available for purchase on BenchChem are specifically designed for in-vitro studies, which are conducted outside of living organisms. In-vitro studies, derived from the Latin term "in glass," involve experiments performed in controlled laboratory settings using cells or tissues. It is important to note that these products are not categorized as medicines or drugs, and they have not received approval from the FDA for the prevention, treatment, or cure of any medical condition, ailment, or disease. We must emphasize that any form of bodily introduction of these products into humans or animals is strictly prohibited by law. It is essential to adhere to these guidelines to ensure compliance with legal and ethical standards in research and experimentation.