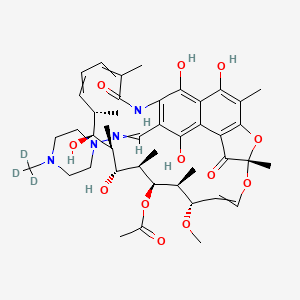
Rifampicin-d3
Overview
Description
Rifampicin-d3 is a deuterated form of rifampicin, an antibiotic used to treat several types of bacterial infections, including tuberculosis, Mycobacterium avium complex, leprosy, and Legionnaires’ disease . The deuterium atoms in this compound replace hydrogen atoms, making it useful in pharmacokinetic studies to track the drug’s metabolism and distribution in the body.
Biochemical Analysis
Biochemical Properties
Rifampicin-d3, like its parent compound rifampicin, is a rifamycin antibiotic and an inhibitor of bacterial RNA polymerase . It inhibits the growth of M. tuberculosis H37Rv in mouse peritoneal macrophages . It also interacts with various species of Staphylococcus, Streptococcus, Haemophilus, and Neisseria .
Cellular Effects
This compound, through its parent compound rifampicin, has significant effects on various types of cells and cellular processes . It influences cell function by affecting cellular functions such as iron acquisition, DNA repair, aerobic respiration, and carbon metabolism .
Molecular Mechanism
This compound shares the same molecular mechanism as rifampicin. It acts by activating the nuclear pregnane X receptor, which in turn affects cytochromes P450, glucuronosyltransferases, and p-glycoprotein activities . This pattern of action may explain many of the rifampicin inducing drug-drug interactions .
Temporal Effects in Laboratory Settings
In laboratory settings, the effects of this compound can change over time . For example, a study showed that the efficacy of rifampicin strongly relies on cellular functions, including iron acquisition, DNA repair, aerobic respiration, and carbon metabolism .
Dosage Effects in Animal Models
In animal models, the effects of this compound can vary with different dosages . For example, a study showed that an intensified high-dose rifampicin-containing regimen significantly improved bactericidal activity for TB meningitis over the first-line, standard-dose rifampicin regimen, without an increase in intracerebral inflammation .
Metabolic Pathways
This compound is involved in several metabolic pathways. For example, it has been found to be associated with pyrimidine, purine, arginine, phenylalanine, tyrosine, and tryptophan metabolic pathways .
Transport and Distribution
This compound, like rifampicin, is transported and distributed within cells and tissues . For example, a study showed that rifampicin can act on a pattern: rifampicin activates the nuclear pregnane X receptor that in turn affects cytochromes P450, glucuronosyltransferases and p-glycoprotein activities .
Subcellular Localization
Given that it shares the same molecular mechanism as rifampicin, it is likely that it also localizes in the same subcellular compartments as rifampicin .
Preparation Methods
Synthetic Routes and Reaction Conditions
Rifampicin-d3 is synthesized starting from rifamycin S and tert-butylamine. The process involves two main steps: formation of rifamycin oxazine and its subsequent ring-opening by 1-amino-4-methyl piperazine . This method has been optimized to improve yield and reduce the use of expensive reagents.
Industrial Production Methods
Industrial production of rifampicin, including its deuterated form, often employs continuous flow synthesis. This method enhances efficiency and reduces costs by coupling reaction steps and purification in a microreactor, achieving a 67% overall yield .
Chemical Reactions Analysis
Types of Reactions
Rifampicin-d3 undergoes various chemical reactions, including:
Oxidation: Rifampicin can be oxidized to form rifampin quinone.
Reduction: Reduction reactions can convert rifampicin to its reduced forms.
Substitution: Substitution reactions involve replacing functional groups on the rifampicin molecule.
Common Reagents and Conditions
Oxidation: Common oxidizing agents include potassium permanganate and hydrogen peroxide.
Reduction: Reducing agents like sodium borohydride are used.
Substitution: Conditions vary depending on the substituent being introduced, often involving nucleophilic reagents.
Major Products
Oxidation: Rifampin quinone.
Reduction: Reduced rifampicin derivatives.
Substitution: Various substituted rifampicin analogs.
Scientific Research Applications
Pharmacokinetic Studies
Rifampicin-d3 as a Tracer in Metabolism Studies
This compound is often employed as a tracer in pharmacokinetic studies to investigate the metabolism and elimination pathways of rifampicin in humans and animal models. The incorporation of deuterium allows for precise tracking of the compound without interference from endogenous substances.
Key Findings
- Metabolic Pathways : Studies have shown that rifampicin undergoes extensive hepatic metabolism primarily through cytochrome P450 enzymes, particularly CYP3A4. This compound can help delineate these pathways by providing a clear distinction between the drug and its metabolites.
- Bioavailability : Research indicates that the bioavailability of rifampicin can vary significantly based on formulation and patient factors. Using this compound allows for more accurate assessments of these variations in clinical settings.
Drug-Drug Interaction Studies
Understanding Interactions with Other Pharmaceuticals
Rifampicin is known for its ability to induce various cytochrome P450 enzymes, leading to significant drug-drug interactions. This compound is utilized in studies to assess these interactions more accurately due to its distinct isotopic signature.
Case Studies
- Combination Therapies : In cases where rifampicin is used alongside other antibiotics (e.g., for treating methicillin-resistant Staphylococcus aureus), this compound has been instrumental in understanding how it alters the pharmacokinetics of co-administered drugs.
- Impact on Vitamin D Metabolism : Research has indicated that rifampicin affects vitamin D metabolism by modulating enzyme activity related to vitamin D hydroxylation. Studies using this compound have clarified these mechanisms, highlighting its role in increasing levels of 25-hydroxyvitamin D .
Clinical Applications
Utilization in Treating Mycobacterial Infections
Rifampicin remains a cornerstone in the treatment of tuberculosis and other mycobacterial infections, with this compound being used in clinical trials to evaluate efficacy and safety profiles.
Treatment Regimens
- Tuberculosis : this compound has been included in clinical trials assessing combination therapies for drug-resistant tuberculosis strains.
- Prophylactic Use : Its effectiveness in eradicating Neisseria meningitidis from carriers' nasopharynxes has also been studied using this compound as part of the regimen.
Application | Description |
---|---|
Tuberculosis Treatment | Evaluated as part of multidrug regimens for enhanced efficacy against resistant strains. |
Prophylaxis for Meningitis | Used to assess effectiveness in preventing transmission among high-risk populations. |
Mechanism of Action
Rifampicin-d3 works by binding to the beta-subunit of microbial DNA-dependent RNA polymerase, inhibiting the enzyme and impeding RNA synthesis . This action reduces the affinity of RNA polymerase for short RNA transcripts, effectively halting bacterial replication. It does not affect mammalian RNA polymerase, making it specific to bacterial cells .
Comparison with Similar Compounds
Similar Compounds
Rifampicin: The non-deuterated form, widely used in clinical settings.
Rifabutin: Another rifamycin antibiotic, used primarily for Mycobacterium avium complex infections.
Rifapentine: Used in the treatment of tuberculosis, with a longer half-life than rifampicin.
Uniqueness
Rifampicin-d3’s deuterium atoms make it particularly useful in pharmacokinetic studies, providing more accurate tracking of the drug’s metabolism and distribution compared to its non-deuterated counterparts .
Biological Activity
Rifampicin-d3, a stable isotopic form of rifampicin, is primarily recognized for its potent antimicrobial properties, particularly against Mycobacterium tuberculosis. This article delves into the biological activity of this compound, focusing on its mechanisms, pharmacokinetics, therapeutic applications, and implications for drug interactions.
This compound exerts its antibacterial effects primarily by inhibiting bacterial RNA synthesis. It binds to the β-subunit of bacterial DNA-dependent RNA polymerase, preventing the transcription of DNA into RNA. This mechanism is crucial in treating infections caused by various bacteria, including those resistant to other antibiotics.
1.1 Interaction with Nuclear Receptors
This compound acts as an agonist for the pregnane X receptor (PXR), which plays a significant role in regulating the expression of cytochrome P450 enzymes involved in drug metabolism. Studies have shown that rifampicin can induce the expression of CYP3A4, significantly affecting the metabolism of co-administered drugs. For instance, a concentration of 20 µM rifampicin increased CYP3A4 mRNA levels by 14-fold in primary human hepatocytes .
2. Pharmacokinetics
The pharmacokinetic profile of this compound reveals significant insights into its absorption, distribution, metabolism, and excretion (ADME). The compound demonstrates high bioavailability and undergoes extensive hepatic metabolism.
Parameter | Value |
---|---|
Bioavailability | ~90% |
Half-life | 2-5 hours |
Volume of distribution | 0.8-1.5 L/kg |
Metabolism | Hepatic (CYP450 enzymes) |
This compound's interaction with PXR not only enhances its own metabolism but also influences the pharmacokinetics of other drugs, leading to potential drug-drug interactions .
3.1 Tuberculosis Treatment
This compound is primarily used in combination therapy for tuberculosis (TB). It is effective against multidrug-resistant strains and has been shown to enhance survival rates in animal models of TB infection .
3.2 Other Infections
Recent studies indicate that rifampicin may also have applications beyond TB:
- Preventive use in surgical settings : Topical application of rifampicin has been shown to significantly reduce the incidence of deep sternal wound infections (DSWI) in patients undergoing coronary artery bypass grafting (CABG) .
- Potential neuroprotective effects : Research suggests that rifampicin could be a candidate for preventing neurodegenerative diseases such as Alzheimer's by restoring autophagy-lysosomal function .
4. Case Studies and Research Findings
Several studies highlight the efficacy and safety profile of this compound:
- In a study involving patients undergoing CABG, the incidence of DSWI dropped from 2.5% to 0.2% with topical rifampicin application .
- A mouse model indicated that rifampicin increases survival rates during TB infection due to its potent antimicrobial activity .
- A study examining vitamin D metabolism revealed that co-treatment with rifampicin and isoniazid enhanced 25-hydroxylation without affecting 1,25-dihydroxyvitamin D levels, indicating its influence on vitamin D metabolism .
5. Conclusion
This compound represents a significant advancement in antibiotic therapy due to its broad-spectrum activity against bacterial pathogens and its role in modulating drug metabolism through PXR activation. Its applications extend beyond tuberculosis treatment, indicating potential uses in surgical prophylaxis and neuroprotection. Ongoing research into its pharmacological properties will further elucidate its capabilities and optimize therapeutic strategies.
Properties
IUPAC Name |
[(7S,11S,12S,13S,14R,15R,16R,17S,18S)-2,15,17,27,29-pentahydroxy-11-methoxy-3,7,12,14,16,18,22-heptamethyl-6,23-dioxo-26-[[4-(trideuteriomethyl)piperazin-1-yl]iminomethyl]-8,30-dioxa-24-azatetracyclo[23.3.1.14,7.05,28]triaconta-1(29),2,4,9,19,21,25,27-octaen-13-yl] acetate | |
---|---|---|
Source | PubChem | |
URL | https://pubchem.ncbi.nlm.nih.gov | |
Description | Data deposited in or computed by PubChem | |
InChI |
InChI=1S/C43H58N4O12/c1-21-12-11-13-22(2)42(55)45-33-28(20-44-47-17-15-46(9)16-18-47)37(52)30-31(38(33)53)36(51)26(6)40-32(30)41(54)43(8,59-40)57-19-14-29(56-10)23(3)39(58-27(7)48)25(5)35(50)24(4)34(21)49/h11-14,19-21,23-25,29,34-35,39,49-53H,15-18H2,1-10H3,(H,45,55)/t21-,23-,24+,25+,29-,34-,35+,39+,43-/m0/s1/i9D3 | |
Source | PubChem | |
URL | https://pubchem.ncbi.nlm.nih.gov | |
Description | Data deposited in or computed by PubChem | |
InChI Key |
JQXXHWHPUNPDRT-LGNOTXDQSA-N | |
Source | PubChem | |
URL | https://pubchem.ncbi.nlm.nih.gov | |
Description | Data deposited in or computed by PubChem | |
Canonical SMILES |
CC1C=CC=C(C(=O)NC2=C(C(=C3C(=C2O)C(=C(C4=C3C(=O)C(O4)(OC=CC(C(C(C(C(C(C1O)C)O)C)OC(=O)C)C)OC)C)C)O)O)C=NN5CCN(CC5)C)C | |
Source | PubChem | |
URL | https://pubchem.ncbi.nlm.nih.gov | |
Description | Data deposited in or computed by PubChem | |
Isomeric SMILES |
[2H]C([2H])([2H])N1CCN(CC1)N=CC2=C3C(=C4C(=C2O)C5=C(C(=C4O)C)O[C@@](C5=O)(OC=C[C@@H]([C@@H]([C@H]([C@@H]([C@@H]([C@@H]([C@H]([C@H](C=CC=C(C(=O)N3)C)C)O)C)O)C)OC(=O)C)C)OC)C)O | |
Source | PubChem | |
URL | https://pubchem.ncbi.nlm.nih.gov | |
Description | Data deposited in or computed by PubChem | |
Molecular Formula |
C43H58N4O12 | |
Source | PubChem | |
URL | https://pubchem.ncbi.nlm.nih.gov | |
Description | Data deposited in or computed by PubChem | |
Molecular Weight |
826.0 g/mol | |
Source | PubChem | |
URL | https://pubchem.ncbi.nlm.nih.gov | |
Description | Data deposited in or computed by PubChem | |
Disclaimer and Information on In-Vitro Research Products
Please be aware that all articles and product information presented on BenchChem are intended solely for informational purposes. The products available for purchase on BenchChem are specifically designed for in-vitro studies, which are conducted outside of living organisms. In-vitro studies, derived from the Latin term "in glass," involve experiments performed in controlled laboratory settings using cells or tissues. It is important to note that these products are not categorized as medicines or drugs, and they have not received approval from the FDA for the prevention, treatment, or cure of any medical condition, ailment, or disease. We must emphasize that any form of bodily introduction of these products into humans or animals is strictly prohibited by law. It is essential to adhere to these guidelines to ensure compliance with legal and ethical standards in research and experimentation.