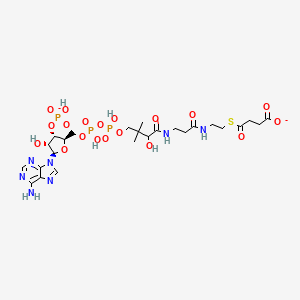
Succinyl coenzyme A sodium salt
- Click on QUICK INQUIRY to receive a quote from our team of experts.
- With the quality product at a COMPETITIVE price, you can focus more on your research.
Overview
Description
Succinyl coenzyme A sodium salt (Succinyl-CoA, CAS 108347-97-3) is a critical intermediate in the tricarboxylic acid (TCA) cycle and heme biosynthesis. Its molecular formula is C₂₅H₃₉N₇NaO₁₉P₃S, with a molecular weight of 889.59 g/mol (varies slightly depending on hydration state) . Structurally, it consists of coenzyme A linked via a thioester bond to succinate.
Preparation Methods
Direct Dissolution of Crystalline Solid
Succinyl-CoA sodium salt is commercially available as a crystalline solid, requiring reconstitution in aqueous buffers for experimental use. This method prioritizes convenience and is widely adopted in laboratory settings .
Solubility and Buffer Compatibility
The solubility of succinyl-CoA sodium salt in phosphate-buffered saline (PBS; pH 7.2) is approximately 10 mg/mL . Dissolution is facilitated by vortexing or brief sonication (30–60 seconds at 25°C). Higher concentrations (up to 50 mg/mL) are achievable in Tris-HCl (pH 8.0) or MOPS-KOH (pH 7.0) buffers, though precipitation risks increase beyond 20 mg/mL .
Table 1: Solubility of Succinyl-CoA Sodium Salt in Common Buffers
Buffer System | pH | Solubility (mg/mL) |
---|---|---|
Phosphate-buffered saline | 7.2 | 10 |
Tris-HCl | 8.0 | 20 |
MOPS-KOH | 7.0 | 15 |
Stability of Aqueous Solutions
Aqueous solutions of succinyl-CoA are highly labile, with degradation rates proportional to temperature and pH. At 4°C, solutions retain 90% integrity for 24 hours but degrade to 60% within 72 hours . For long-term storage, aliquots are recommended to be frozen at -80°C, though repeated freeze-thaw cycles accelerate hydrolysis .
Preparation of Stock Solutions and In Vivo Formulations
Stock solutions of succinyl-CoA sodium salt are prerequisite for enzymatic assays and metabolic studies. GlpBio’s standardized protocols provide a framework for preparing solutions of defined molarity and compatibility with in vivo systems .
Molarity Calculations and Dilutions
The molecular weight of succinyl-CoA sodium salt (867.6 g/mol) is used to calculate molarity. For example, a 10 mM solution requires dissolving 8.68 mg of the compound in 1 mL of buffer .
Table 2: Stock Solution Preparation Guide
Mass (mg) | Volume for 1 mM (mL) | Volume for 5 mM (mL) | Volume for 10 mM (mL) |
---|---|---|---|
1 | 1.15 | 0.23 | 0.12 |
5 | 5.76 | 1.15 | 0.58 |
10 | 11.53 | 2.31 | 1.15 |
In Vivo Formulation Strategies
For animal studies, succinyl-CoA is often dissolved in dimethyl sulfoxide (DMSO) to enhance permeability, followed by dilution in biocompatible solvents like PEG300 and Tween 80. A typical formulation involves:
-
Dissolving 10 mg of succinyl-CoA in 100 μL DMSO (100 mg/mL master stock).
-
Mixing with 300 μL PEG300 and 50 μL Tween 80.
This protocol ensures clarity and stability for intraperitoneal or intravenous administration.
Analytical Techniques for Purity and Concentration
Quality control is paramount to validate succinyl-CoA preparations. UV-Vis spectroscopy and HPLC are the gold standards for assessing purity and concentration .
UV-Vis Spectrophotometry
The absorbance maximum of succinyl-CoA at 258 nm (ε = 16.4 mM⁻¹cm⁻¹) allows direct quantification. A 1:100 dilution in PBS typically yields an absorbance of 0.164 at 258 nm for a 1 mM solution .
High-Performance Liquid Chromatography
Reverse-phase HPLC with a C18 column (gradient: 5–35% acetonitrile in 0.1% trifluoroacetic acid over 20 minutes) resolves succinyl-CoA from contaminants. Retention times range from 12–14 minutes, with peak area integration used to calculate purity (≥70% acceptable for most applications) .
Troubleshooting Common Preparation Challenges
Low Solubility in Aqueous Buffers
If precipitation occurs during dissolution, incremental addition of 0.1 N NaOH (up to 10 μL per mL) can enhance solubility without altering pH significantly . Alternatively, switching to a high-ionic-strength buffer like HEPES-NaOH (pH 7.5) improves dissolution .
Chemical Reactions Analysis
Types of Reactions
Succinyl-Coenzyme A (sodium salt) undergoes several types of chemical reactions, including:
Hydrolysis: The hydrolytic release of coenzyme A by succinyl-CoA synthetase (succinate thiokinase) to form succinate.
Condensation: Combines with glycine to form δ-aminolevulinic acid (dALA) in the first step of porphyrin and heme synthesis.
Common Reagents and Conditions
Hydrolysis: Typically occurs in the presence of succinyl-CoA synthetase under physiological conditions.
Condensation: Requires the enzyme ALA synthase and occurs in the mitochondria.
Major Products
Succinate: Formed through the hydrolysis of succinyl-CoA.
δ-Aminolevulinic Acid (dALA): Formed through the condensation reaction with glycine.
Scientific Research Applications
Scientific Research Applications
-
Metabolic Studies
- Succinyl coenzyme A sodium salt plays a significant role in the citric acid cycle, where it is converted to succinate, facilitating substrate-level phosphorylation. This reaction is essential for the generation of energy in aerobic metabolism .
- It is also involved in heme biosynthesis and ketone body activation, particularly during anaerobic growth conditions .
- Enzyme Kinetics and Specificity
- Protein Succinylation
- Biosynthetic Pathways
Case Study 1: Enzymatic Activity Assessment
A study investigated the activity of succinyl coenzyme A synthetase in various organisms. The enzyme catalyzes the reversible conversion of succinyl coenzyme A to succinate, yielding nucleoside triphosphates during aerobic metabolism. The findings indicated that this enzyme acts as a regulatory point within the citric acid cycle, demonstrating its importance in metabolic control .
Case Study 2: Clinical Implications of Succinyl CoA Deficiency
Research on succinyl-CoA:3-ketoacid CoA transferase deficiency revealed significant clinical variability among patients. The study emphasized the role of succinyl coenzyme A in metabolic pathways and highlighted how mutations affecting its metabolism could lead to severe metabolic crises. Management strategies included dietary restrictions and carnitine supplementation, showcasing the compound's relevance in clinical settings .
Data Table: Applications of this compound
Application Area | Description | Key Findings/Implications |
---|---|---|
Metabolic Studies | Involved in the citric acid cycle; energy production | Essential for ATP generation via substrate-level phosphorylation |
Enzyme Kinetics | Substrate for studying enzyme specificity | Insights into enzyme mechanisms and metabolic regulation |
Protein Succinylation | Non-enzymatic modification of lysine residues | Impacts protein function and stability |
Biosynthetic Pathways | Substrate for adipic acid production in E. coli | Importance in synthetic biology applications |
Clinical Implications | Role in metabolic disorders related to succinyl CoA metabolism | Highlights need for dietary management strategies |
Mechanism of Action
Succinyl-Coenzyme A (sodium salt) exerts its effects primarily through its role in the citric acid cycle. It is formed by the decarboxylation of α-ketoglutarate and is subsequently converted to succinate by succinyl-CoA synthetase. This conversion is coupled with the generation of guanosine triphosphate (GTP) or ATP, which are essential for cellular energy metabolism . Additionally, succinyl-CoA is involved in the synthesis of heme, where it combines with glycine to form δ-aminolevulinic acid .
Comparison with Similar Compounds
Key Roles:
- TCA Cycle : Generated from α-ketoglutarate via α-ketoglutarate dehydrogenase, then converted to succinate by succinyl-CoA synthetase .
- Heme Synthesis: Combines with glycine to form δ-aminolevulinic acid (ALA), a precursor of porphyrins .
- Ketone Body Metabolism : Involved in ketone oxidation in extrahepatic tissues .
- Non-Enzymatic Modifications: Acts as a succinyl donor for lysine succinylation in proteins .
Physicochemical Properties :
- Purity : ≥85% (commercial preparations) .
- Solubility : 50 mg/mL in water .
- Storage : Stable at −20°C in powder form .
Comparison with Similar Acyl-CoA Derivatives
Acyl-coenzyme A (CoA) thioesters share structural similarities but differ in metabolic roles, enzymatic interactions, and applications. Below is a detailed comparison:
Table 1: Structural and Functional Comparison of Acyl-CoA Derivatives
Key Differences:
Metabolic Pathways :
- Succinyl-CoA : Central to energy production (TCA cycle) and heme biosynthesis .
- Acetyl-CoA : Primarily involved in lipid synthesis and acetylation reactions (e.g., histone modification) .
- Malonyl-CoA : Inhibits carnitine palmitoyltransferase-1 (CPT1), regulating fatty acid oxidation .
Enzymatic Specificity :
- Succinyl-CoA synthetase catalyzes ATP-dependent conversion of succinyl-CoA to succinate, a reversible reaction .
- Acetyl-CoA synthetase activates acetate for lipid synthesis, requiring AMP instead of ATP .
Non-Enzymatic Reactivity: Succinyl-CoA uniquely undergoes non-enzymatic reactions with lysine residues in proteins, a process critical for post-translational modifications . Other acyl-CoAs (e.g., acetyl-CoA) primarily rely on enzymatic catalysis for such modifications .
Table 2: Key Studies on Succinyl-CoA and Related Compounds
Biological Activity
Succinyl coenzyme A (Succinyl-CoA) sodium salt is a pivotal metabolite in various biological processes, particularly in the citric acid cycle and heme synthesis. This article explores its biological activity, applications, and relevant research findings.
Succinyl-CoA sodium salt is a coenzyme A derivative with the molecular formula C25H40N7O19P3S and a molecular weight of 867.6 g/mol. It is typically supplied as a crystalline solid and is soluble in aqueous buffers, with a solubility of approximately 10 mg/ml in phosphate-buffered saline (PBS) at pH 7.2 .
Role in Metabolism
1. Citric Acid Cycle:
Succinyl-CoA plays a critical role in the citric acid cycle (Krebs cycle), where it is converted to succinate by succinyl-CoA synthetase. This reaction is notable for being one of the few substrate-level phosphorylation events in the cycle, generating GTP or ATP depending on the organism .
2. Heme Biosynthesis:
Succinyl-CoA is essential for the synthesis of δ-aminolevulinic acid (ALA), which is the first step in heme production. This pathway underscores its importance in both energy metabolism and the synthesis of vital biomolecules .
Biological Activities
1. Enzymatic Reactions:
Succinyl-CoA serves as a substrate for various enzymes, including:
- 5-Aminolevulinate Synthase (ALA Synthase): Catalyzes the condensation of glycine and Succinyl-CoA to form ALA.
- Acetate:Succinate CoA-Transferase: Involved in anaerobic metabolism, particularly in organisms like Fasciola hepatica, facilitating acetate and propionate production .
2. Non-Enzymatic Succinylation:
It has been utilized for nonenzymatic succinylation of lysine residues in proteins, which can influence protein function and stability .
Table 1: Summary of Key Studies Involving Succinyl-CoA
Notable Findings
- Neuromotor Dysfunction: Deficiency in Succinyl-CoA can lead to significant health issues such as growth retardation and loss of motor skills due to impaired heme synthesis .
- Enzyme Specificity: Research indicates that succinyl-CoA synthetases exhibit varied nucleotide specificity across different organisms, impacting metabolic efficiency .
Applications
Succinyl-CoA sodium salt has diverse applications in biochemical research:
- Metabolic Studies: Used to investigate metabolic pathways related to porphyrias and other metabolic disorders .
- Biochemical Assays: Serves as a substrate for studying enzyme kinetics and specificity in various metabolic reactions .
- Protein Modification: Employed for succinylating proteins to study post-translational modifications and their effects on protein function.
Q & A
Basic Research Questions
Q. What is the biochemical role of succinyl coenzyme A sodium salt in the citric acid cycle and heme synthesis?
Succinyl coenzyme A (succinyl-CoA) is a critical intermediate in the citric acid cycle, where it is generated via α-ketoglutarate dehydrogenase-mediated decarboxylation of α-ketoglutarate. It is subsequently converted to succinate by succinyl-CoA synthetase, coupled with GTP/ATP production . In heme biosynthesis, succinyl-CoA combines with glycine via 5-aminolevulinate synthase (ALAS) to form δ-aminolevulinic acid (ALA), the rate-limiting step . Researchers studying these pathways should employ enzymatic assays (e.g., spectrophotometric monitoring of NADH/NADPH oxidation) or stable isotope tracing to validate flux through these pathways.
Q. How should succinyl-CoA sodium salt be prepared and stored to ensure stability in experimental settings?
Succinyl-CoA sodium salt is hygroscopic and degrades rapidly at room temperature. For optimal stability, reconstitute lyophilized powder in ultrapure water (50 mg/mL) and aliquot into single-use portions to avoid freeze-thaw cycles. Store at −20°C in amber vials to minimize light and moisture exposure . Purity (>85%–95%) should be verified via HPLC or mass spectrometry before use, as impurities (e.g., free CoA) can interfere with enzyme kinetics .
Q. What methods are recommended for quantifying succinyl-CoA in cellular extracts?
Quantification can be achieved via:
- Enzymatic assays : Couple succinyl-CoA to succinate conversion with succinyl-CoA synthetase, measuring GTP production via luciferase-based systems .
- NMR spectroscopy : Use ¹H NMR with deuterated solvents and internal standards (e.g., TSP) to resolve succinyl-CoA peaks at ~2.5 ppm (methylene protons) .
- LC-MS/MS : Employ reverse-phase chromatography with electrospray ionization (ESI) and monitor the transition m/z 867.6 → 428.2 (characteristic fragment) .
Advanced Research Questions
Q. How can succinyl-CoA sodium salt be used to study non-enzymatic protein succinylation, and what controls are essential?
Succinyl-CoA serves as a substrate for non-enzymatic lysine succinylation, a post-translational modification implicated in metabolic regulation. To study this:
- Incubate purified proteins (e.g., αB-crystallin) with succinyl-CoA (1–5 mM) in buffers mimicking physiological pH (7.4) and temperature (37°C) .
- Include controls with acetyl-CoA or malonyl-CoA to assess specificity.
- Validate modifications via Western blot using anti-succinyllysine antibodies or mass spectrometry .
Q. What experimental strategies resolve contradictions in succinyl-CoA’s role in metabolic diseases (e.g., vitamin B12 deficiency)?
Vitamin B12 deficiency reduces succinyl-CoA availability, impairing heme synthesis and causing neuromotor dysfunction . To address conflicting
- Use isotopic tracing (¹³C-glucose or ¹³C-propionate) in B12-deficient cell models to track succinyl-CoA flux into heme versus the citric acid cycle .
- Compare transcriptomic (ALAS expression) and metabolomic (ALA, heme levels) profiles under varying CoA availability .
- Control for mitochondrial dysfunction (e.g., measure ATP/ROS levels) to disentangle primary vs. secondary effects .
Q. How do purity variations in commercial succinyl-CoA sodium salt impact enzyme kinetic studies?
Commercial preparations vary in purity (≥85% to >95%), which can skew kinetic parameters. For example, residual acetyl-CoA in low-purity batches may compete with succinyl-CoA in assays like citrate synthase or 5-aminolevulinate synthase . Mitigate this by:
- Pre-treating samples with acyl-CoA hydrolases to degrade contaminants.
- Validating purity via HPLC-UV (260 nm for CoA derivatives) .
- Using high-purity batches (>95%) for precise Kₘ and Vₘₐₓ calculations .
Q. What computational models are used to predict succinyl-CoA’s interaction with enzymes like succinate dehydrogenase (SDH)?
Molecular docking (e.g., AutoDock Vina) and molecular dynamics simulations can model succinyl-CoA binding to SDH. Key steps include:
- Retrieving SDH crystal structures (PDB: 2WX2) and optimizing succinyl-CoA’s charge states via quantum mechanics (QM) .
- Simulating binding under physiological ionic strength (150 mM KCl) and pH (7.4) .
- Validating predictions with mutagenesis (e.g., His-His motifs in the active site) .
Q. Methodological Considerations
Q. How to troubleshoot low recovery of succinyl-CoA in cell lysates?
Low recovery often stems from rapid degradation by endogenous hydrolases. To improve yields:
- Add 10 mM iodoacetamide to lysate buffers to inhibit thioesterases .
- Use ice-cold perchloric acid (6% v/v) for immediate metabolite extraction .
- Include stabilizers like 1 mM dithiothreitol (DTT) to prevent CoA oxidation .
Q. What are the limitations of using succinyl-CoA sodium salt in in vitro models of mitochondrial dysfunction?
Exogenous succinyl-CoA cannot cross mitochondrial membranes, limiting its utility in intact-cell studies. Alternatives include:
Properties
CAS No. |
108347-97-3 |
---|---|
Molecular Formula |
C25H40N7NaO19P3S |
Molecular Weight |
890.6 g/mol |
IUPAC Name |
sodium;4-[2-[3-[[4-[[[(2R,3S,4R,5R)-5-(6-aminopurin-9-yl)-4-hydroxy-3-phosphonooxyoxolan-2-yl]methoxy-hydroxyphosphoryl]oxy-hydroxyphosphoryl]oxy-2-hydroxy-3,3-dimethylbutanoyl]amino]propanoylamino]ethylsulfanyl]-4-oxobutanoate |
InChI |
InChI=1S/C25H40N7O19P3S.Na/c1-25(2,20(38)23(39)28-6-5-14(33)27-7-8-55-16(36)4-3-15(34)35)10-48-54(45,46)51-53(43,44)47-9-13-19(50-52(40,41)42)18(37)24(49-13)32-12-31-17-21(26)29-11-30-22(17)32;/h11-13,18-20,24,37-38H,3-10H2,1-2H3,(H,27,33)(H,28,39)(H,34,35)(H,43,44)(H,45,46)(H2,26,29,30)(H2,40,41,42);/t13-,18-,19-,20+,24-;/m1./s1 |
InChI Key |
MZSOFLIDFHDGAA-YXKORUMSSA-N |
SMILES |
CC(C)(COP(=O)(O)OP(=O)(O)OCC1C(C(C(O1)N2C=NC3=C(N=CN=C32)N)O)OP(=O)(O)[O-])C(C(=O)NCCC(=O)NCCSC(=O)CCC(=O)[O-])O |
Isomeric SMILES |
CC(C)(COP(=O)(O)OP(=O)(O)OC[C@@H]1[C@H]([C@H]([C@@H](O1)N2C=NC3=C(N=CN=C32)N)O)OP(=O)(O)O)[C@H](C(=O)NCCC(=O)NCCSC(=O)CCC(=O)O)O.[Na] |
Canonical SMILES |
CC(C)(COP(=O)(O)OP(=O)(O)OCC1C(C(C(O1)N2C=NC3=C(N=CN=C32)N)O)OP(=O)(O)O)C(C(=O)NCCC(=O)NCCSC(=O)CCC(=O)O)O.[Na] |
Origin of Product |
United States |
Disclaimer and Information on In-Vitro Research Products
Please be aware that all articles and product information presented on BenchChem are intended solely for informational purposes. The products available for purchase on BenchChem are specifically designed for in-vitro studies, which are conducted outside of living organisms. In-vitro studies, derived from the Latin term "in glass," involve experiments performed in controlled laboratory settings using cells or tissues. It is important to note that these products are not categorized as medicines or drugs, and they have not received approval from the FDA for the prevention, treatment, or cure of any medical condition, ailment, or disease. We must emphasize that any form of bodily introduction of these products into humans or animals is strictly prohibited by law. It is essential to adhere to these guidelines to ensure compliance with legal and ethical standards in research and experimentation.