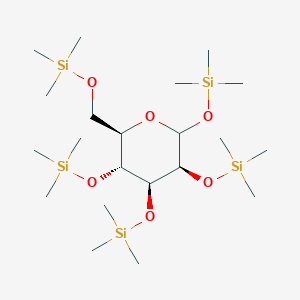
D-Mannopyranose, 1,2,3,4,6-pentakis-O-(trimethylsilyl)-
- Click on QUICK INQUIRY to receive a quote from our team of experts.
- With the quality product at a COMPETITIVE price, you can focus more on your research.
Overview
Description
Synthesis Analysis
The synthesis of D-Mannopyranose derivatives, including those with trimethylsilyl groups, typically involves selective protection and deprotection strategies. For instance, synthesis approaches for related compounds have utilized protective groups and methylation with diazomethane to achieve targeted structural features (Seymour, 1973). Chemical synthesis strategies often aim to introduce specific functional groups or structural modifications while preserving the integrity of the carbohydrate skeleton.
Molecular Structure Analysis
The molecular structure of D-Mannopyranose derivatives, such as those analyzed by gas-liquid chromatography-chemical-ionization mass spectrometry, reveals distinct fragmentation patterns and high-intensity fragment ions, facilitating the differentiation between various isomers and derivatives (Murata & Takahashi, 1978). The structural analysis underscores the importance of trimethylsilyl groups in enhancing the detectability and analysis of carbohydrate molecules.
Chemical Reactions and Properties
The chemical reactivity of D-Mannopyranose derivatives is influenced by the presence of trimethylsilyl groups. These groups can be selectively introduced or removed, altering the compound's reactivity and properties. The synthesis of complex oligosaccharides from D-Mannose, involving dehydrative glycosylation reactions, highlights the compound's utility in forming glycosidic bonds and constructing carbohydrate structures (Hirooka et al., 2002).
Scientific Research Applications
Synthesis of Hexopyranose Analogs : Research by Darrow and Drueckhammer (1994) demonstrates the synthesis of cyclic analogs of D-glucopyranose and D-mannopyranose, where the anomeric carbon is replaced with phosphorus. These analogs have applications in studying carbohydrate chemistry and reactions (Darrow & Drueckhammer, 1994).
Stereoselective C-Allylation : Hosomi, Sakata, and Sakurai (1984) focused on the highly stereoselective C-allylation of glycopyranosides, including D-mannopyranose derivatives. This method has applications in the synthesis of complex carbohydrate structures (Hosomi, Sakata, & Sakurai, 1984).
Carbasugar Synthesis : Gómez et al. (2004) developed a method for synthesizing carbasugars from D-mannose, which are important in the study of glycosidase inhibitors and the development of new therapeutic agents (Gómez, Moreno, Valverde, & López, 2004).
Process Improvement in Mannose Derivatives Synthesis : Wang We (2014) modified existing synthetic methods for important mannose derivatives, demonstrating the efficiency of microwave-assisted synthesis. This has implications for the industrial production of these compounds (Wang We, 2014).
Mass Spectrometry of Pyranoses : Havlicek, Brennan, and Scheuer (1971) studied the influence of stereochemistry on the mass spectra of trimethylsilylated pyranoses, including D-mannopyranose. This research aids in the structural analysis of carbohydrates (Havlicek, Brennan, & Scheuer, 1971).
Future Directions
The future directions for “D-Mannopyranose, 1,2,3,4,6-pentakis-O-(trimethylsilyl)-” are not specified in the search results. However, given its structural similarity to D-Mannopyranose, it may find use in similar applications, such as in the study of T-lymphocyte mediated inflammatory diseases .
properties
IUPAC Name |
trimethyl-[[(2R,3R,4S,5S)-3,4,5,6-tetrakis(trimethylsilyloxy)oxan-2-yl]methoxy]silane |
Source
|
---|---|---|
Source | PubChem | |
URL | https://pubchem.ncbi.nlm.nih.gov | |
Description | Data deposited in or computed by PubChem | |
InChI |
InChI=1S/C21H52O6Si5/c1-28(2,3)22-16-17-18(24-29(4,5)6)19(25-30(7,8)9)20(26-31(10,11)12)21(23-17)27-32(13,14)15/h17-21H,16H2,1-15H3/t17-,18-,19+,20+,21?/m1/s1 |
Source
|
Source | PubChem | |
URL | https://pubchem.ncbi.nlm.nih.gov | |
Description | Data deposited in or computed by PubChem | |
InChI Key |
PPFHNIVPOLWPCF-AUGMSIGLSA-N |
Source
|
Source | PubChem | |
URL | https://pubchem.ncbi.nlm.nih.gov | |
Description | Data deposited in or computed by PubChem | |
Canonical SMILES |
C[Si](C)(C)OCC1C(C(C(C(O1)O[Si](C)(C)C)O[Si](C)(C)C)O[Si](C)(C)C)O[Si](C)(C)C |
Source
|
Source | PubChem | |
URL | https://pubchem.ncbi.nlm.nih.gov | |
Description | Data deposited in or computed by PubChem | |
Isomeric SMILES |
C[Si](C)(C)OC[C@@H]1[C@H]([C@@H]([C@@H](C(O1)O[Si](C)(C)C)O[Si](C)(C)C)O[Si](C)(C)C)O[Si](C)(C)C |
Source
|
Source | PubChem | |
URL | https://pubchem.ncbi.nlm.nih.gov | |
Description | Data deposited in or computed by PubChem | |
Molecular Formula |
C21H52O6Si5 |
Source
|
Source | PubChem | |
URL | https://pubchem.ncbi.nlm.nih.gov | |
Description | Data deposited in or computed by PubChem | |
Molecular Weight |
541.1 g/mol |
Source
|
Source | PubChem | |
URL | https://pubchem.ncbi.nlm.nih.gov | |
Description | Data deposited in or computed by PubChem | |
Product Name |
D-Mannopyranose, 1,2,3,4,6-pentakis-O-(trimethylsilyl)- |
Disclaimer and Information on In-Vitro Research Products
Please be aware that all articles and product information presented on BenchChem are intended solely for informational purposes. The products available for purchase on BenchChem are specifically designed for in-vitro studies, which are conducted outside of living organisms. In-vitro studies, derived from the Latin term "in glass," involve experiments performed in controlled laboratory settings using cells or tissues. It is important to note that these products are not categorized as medicines or drugs, and they have not received approval from the FDA for the prevention, treatment, or cure of any medical condition, ailment, or disease. We must emphasize that any form of bodily introduction of these products into humans or animals is strictly prohibited by law. It is essential to adhere to these guidelines to ensure compliance with legal and ethical standards in research and experimentation.