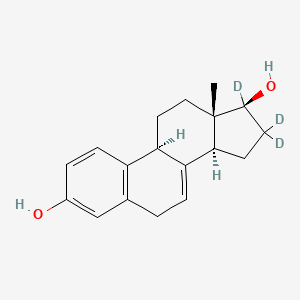
17beta-Dihydro Equilin-16,16,17-d3
- Click on QUICK INQUIRY to receive a quote from our team of experts.
- With the quality product at a COMPETITIVE price, you can focus more on your research.
Overview
Description
17beta-Dihydro Equilin-16,16,17-d3 is a deuterium-labeled compound, which is a stable isotope of the estrogenic steroid equilin. Equilin is one of the estrogens present in the mixture of estrogens isolated from horse urine and marketed as Premarin. The compound has a molecular formula of C18H19D3O2 and a molecular weight of 273.38 .
Preparation Methods
Synthetic Routes and Reaction Conditions
The synthesis of 17beta-Dihydro Equilin-16,16,17-d3 involves the incorporation of deuterium atoms at specific positions in the equilin molecule. This can be achieved through various synthetic routes, including catalytic hydrogenation using deuterium gas or deuterated reagents. The reaction conditions typically involve the use of a catalyst such as palladium on carbon (Pd/C) under an inert atmosphere .
Industrial Production Methods
Industrial production of this compound follows similar synthetic routes but on a larger scale. The process involves stringent quality control measures to ensure the purity and isotopic enrichment of the final product. The compound is often produced as a reference standard for analytical purposes .
Chemical Reactions Analysis
Types of Reactions
17beta-Dihydro Equilin-16,16,17-d3 undergoes various chemical reactions, including:
Oxidation: The compound can be oxidized to form quinones and other oxidation products.
Reduction: Reduction reactions can convert the compound to its corresponding dihydro derivatives.
Substitution: The compound can undergo substitution reactions, particularly at the hydroxyl groups.
Common Reagents and Conditions
Oxidation: Common oxidizing agents include potassium permanganate (KMnO4) and chromium trioxide (CrO3).
Reduction: Reducing agents such as lithium aluminum hydride (LiAlH4) and sodium borohydride (NaBH4) are commonly used.
Substitution: Reagents such as alkyl halides and acyl chlorides can be used for substitution reactions.
Major Products Formed
The major products formed from these reactions include various hydroxylated and deuterated derivatives of equilin, which can be further analyzed for their biological and chemical properties .
Scientific Research Applications
17beta-Dihydro Equilin-16,16,17-d3 is widely used in scientific research due to its stable isotope labeling. Some of its applications include:
Chemistry: Used as a reference standard in analytical chemistry for method development and validation.
Biology: Employed in metabolic studies to trace the pathways and transformations of equilin in biological systems.
Medicine: Used in pharmacokinetic studies to understand the absorption, distribution, metabolism, and excretion of equilin.
Industry: Utilized in the quality control of pharmaceutical products containing equilin.
Mechanism of Action
The mechanism of action of 17beta-Dihydro Equilin-16,16,17-d3 is similar to that of equilin. It acts as an estrogen receptor agonist, binding to estrogen receptors in target tissues. This binding activates the transcription of estrogen-responsive genes, leading to various physiological effects such as the regulation of reproductive functions and secondary sexual characteristics .
Comparison with Similar Compounds
Similar Compounds
Equilin: The parent compound, which is not deuterated.
17alpha-Dihydro Equilin: Another isomer of equilin with different biological activity.
17beta-Dihydro Equilenin: A related compound with similar estrogenic activity.
Uniqueness
17beta-Dihydro Equilin-16,16,17-d3 is unique due to its deuterium labeling, which provides enhanced stability and allows for precise tracing in metabolic studies. This makes it a valuable tool in research applications where isotopic labeling is required .
Properties
IUPAC Name |
(9S,13S,14S,17S)-16,16,17-trideuterio-13-methyl-6,9,11,12,14,15-hexahydrocyclopenta[a]phenanthrene-3,17-diol |
Source
|
---|---|---|
Source | PubChem | |
URL | https://pubchem.ncbi.nlm.nih.gov | |
Description | Data deposited in or computed by PubChem | |
InChI |
InChI=1S/C18H22O2/c1-18-9-8-14-13-5-3-12(19)10-11(13)2-4-15(14)16(18)6-7-17(18)20/h3-5,10,14,16-17,19-20H,2,6-9H2,1H3/t14-,16+,17+,18+/m1/s1/i7D2,17D |
Source
|
Source | PubChem | |
URL | https://pubchem.ncbi.nlm.nih.gov | |
Description | Data deposited in or computed by PubChem | |
InChI Key |
NLLMJANWPUQQTA-XPOHSFSVSA-N |
Source
|
Source | PubChem | |
URL | https://pubchem.ncbi.nlm.nih.gov | |
Description | Data deposited in or computed by PubChem | |
Canonical SMILES |
CC12CCC3C(=CCC4=C3C=CC(=C4)O)C1CCC2O |
Source
|
Source | PubChem | |
URL | https://pubchem.ncbi.nlm.nih.gov | |
Description | Data deposited in or computed by PubChem | |
Isomeric SMILES |
[2H][C@]1([C@]2(CC[C@H]3C(=CCC4=C3C=CC(=C4)O)[C@@H]2CC1([2H])[2H])C)O |
Source
|
Source | PubChem | |
URL | https://pubchem.ncbi.nlm.nih.gov | |
Description | Data deposited in or computed by PubChem | |
Molecular Formula |
C18H22O2 |
Source
|
Source | PubChem | |
URL | https://pubchem.ncbi.nlm.nih.gov | |
Description | Data deposited in or computed by PubChem | |
Molecular Weight |
273.4 g/mol |
Source
|
Source | PubChem | |
URL | https://pubchem.ncbi.nlm.nih.gov | |
Description | Data deposited in or computed by PubChem | |
Disclaimer and Information on In-Vitro Research Products
Please be aware that all articles and product information presented on BenchChem are intended solely for informational purposes. The products available for purchase on BenchChem are specifically designed for in-vitro studies, which are conducted outside of living organisms. In-vitro studies, derived from the Latin term "in glass," involve experiments performed in controlled laboratory settings using cells or tissues. It is important to note that these products are not categorized as medicines or drugs, and they have not received approval from the FDA for the prevention, treatment, or cure of any medical condition, ailment, or disease. We must emphasize that any form of bodily introduction of these products into humans or animals is strictly prohibited by law. It is essential to adhere to these guidelines to ensure compliance with legal and ethical standards in research and experimentation.