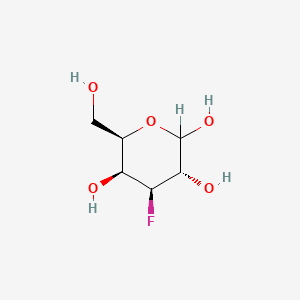
3-Deoxy-3-fluoro-D-galactose
- Click on QUICK INQUIRY to receive a quote from our team of experts.
- With the quality product at a COMPETITIVE price, you can focus more on your research.
Overview
Description
3-Deoxy-3-fluoro-D-galactose is a fluorinated carbohydrate used primarily in glycobiology research. Glycobiology studies the structure, synthesis, biology, and evolution of sugars. This compound is particularly valuable for its ability to mimic natural carbohydrates while offering enhanced stability and unique properties due to the presence of fluorine .
Mechanism of Action
Target of Action
3-Deoxy-3-fluoro-D-galactose (3DFGal) primarily targets the metabolic pathway of gram-positive pathogens . It is used as a tool for studying the enzymatic reaction catalyzed by galactokinase , which is involved in the conversion of 3DFGal to D-galactose .
Mode of Action
The interaction of 3DFGal with its targets results in a shift in the metabolic pathway of gram-positive pathogens from glycolysis to gluconeogenesis . This shift is facilitated by the compound’s structural similarity to D-galactose, allowing it to bind to the same enzymes and receptors .
Biochemical Pathways
3DFGal affects the glycolysis and gluconeogenesis pathways. By mimicking D-galactose, it competes for the same enzymes involved in these pathways . This competition results in a shift from glycolysis, the breakdown of glucose for energy, to gluconeogenesis, the production of glucose from non-carbohydrate sources .
Result of Action
The primary result of 3DFGal’s action is the shift in metabolic pathways in gram-positive pathogens . This shift can potentially disrupt the normal metabolic processes of these pathogens, inhibiting their growth and proliferation .
Action Environment
The action of 3DFGal can be influenced by various environmental factors. For instance, the pH of the environment can affect the rate of 3DFGal hydrolysis . Furthermore, the presence of other sugars, such as glucose, could potentially affect the compound’s efficacy by competing for the same metabolic pathways . .
Biochemical Analysis
Biochemical Properties
3-Deoxy-3-fluoro-D-galactose interacts with various enzymes and proteins in biochemical reactions . It is utilized in biomedicine for its potent inhibitory properties against enzymes involved in carbohydrate metabolism
Cellular Effects
It is known to influence cell function, including impacts on cell signaling pathways, gene expression, and cellular metabolism .
Molecular Mechanism
The molecular mechanism of this compound is complex. It exerts its effects at the molecular level, including binding interactions with biomolecules, enzyme inhibition or activation, and changes in gene expression
Metabolic Pathways
This compound is involved in several metabolic pathways. It has been shown to shift the metabolic pathway of gram-positive pathogens from glycolysis to gluconeogenesis . It interacts with various enzymes and cofactors, and may also have effects on metabolic flux or metabolite levels .
Transport and Distribution
This compound is transported into tissues at rates similar to those of glucose . It is distributed within cells and tissues, potentially interacting with transporters or binding proteins .
Preparation Methods
Synthetic Routes and Reaction Conditions
The synthesis of 3-Deoxy-3-fluoro-D-galactose typically involves the fluorination of D-galactose derivatives. One common method starts with 1,2:5,6-di-O-isopropylidene-α-D-gulofuranose, which undergoes a series of reactions including sulfonation and fluorination to yield the desired product . The reaction conditions often involve the use of tetra-alkylammonium fluoride in dipolar aprotic solvents like methyl cyanide .
Industrial Production Methods
While specific industrial production methods for this compound are not widely documented, the general approach involves scaling up the laboratory synthesis methods. This includes optimizing reaction conditions for higher yields and purity, and employing industrial-scale reactors and purification systems.
Chemical Reactions Analysis
Types of Reactions
3-Deoxy-3-fluoro-D-galactose can undergo various chemical reactions, including:
Oxidation: Typically involves reagents like potassium permanganate or chromium trioxide.
Reduction: Can be achieved using reducing agents such as sodium borohydride.
Substitution: Fluorine atoms can be substituted with other functional groups using nucleophilic substitution reactions.
Common Reagents and Conditions
Oxidation: Potassium permanganate in an acidic medium.
Reduction: Sodium borohydride in methanol.
Substitution: Tetra-alkylammonium fluoride in dipolar aprotic solvents.
Major Products Formed
The major products formed from these reactions depend on the specific conditions and reagents used. For example, oxidation can yield carboxylic acids, while reduction typically produces alcohols. Substitution reactions can introduce various functional groups, enhancing the compound’s versatility in research applications .
Scientific Research Applications
3-Deoxy-3-fluoro-D-galactose has a wide range of applications in scientific research:
Chemistry: Used as a chemical probe to study molecular recognition and carbohydrate-protein interactions.
Biology: Helps in understanding glycan formation and degradation, and protein-glycan recognition.
Industry: Used in the development of novel materials with unique properties due to the presence of fluorine.
Comparison with Similar Compounds
Similar Compounds
- 3-Deoxy-3-fluoro-D-glucose
- 3-Deoxy-3-fluoro-L-fucose
- 2-Deoxy-2-fluoro-D-glucose
Comparison
Compared to these similar compounds, 3-Deoxy-3-fluoro-D-galactose offers unique advantages due to its specific structural features. The presence of fluorine at the 3-position provides enhanced stability and unique reactivity, making it particularly useful in glycobiology research .
Properties
IUPAC Name |
(3S,4S,5S,6R)-4-fluoro-6-(hydroxymethyl)oxane-2,3,5-triol |
Source
|
---|---|---|
Source | PubChem | |
URL | https://pubchem.ncbi.nlm.nih.gov | |
Description | Data deposited in or computed by PubChem | |
InChI |
InChI=1S/C6H11FO5/c7-3-4(9)2(1-8)12-6(11)5(3)10/h2-6,8-11H,1H2/t2-,3+,4+,5-,6?/m1/s1 |
Source
|
Source | PubChem | |
URL | https://pubchem.ncbi.nlm.nih.gov | |
Description | Data deposited in or computed by PubChem | |
InChI Key |
BUMRBAMACDBPKO-SVZMEOIVSA-N |
Source
|
Source | PubChem | |
URL | https://pubchem.ncbi.nlm.nih.gov | |
Description | Data deposited in or computed by PubChem | |
Canonical SMILES |
C(C1C(C(C(C(O1)O)O)F)O)O |
Source
|
Source | PubChem | |
URL | https://pubchem.ncbi.nlm.nih.gov | |
Description | Data deposited in or computed by PubChem | |
Isomeric SMILES |
C([C@@H]1[C@@H]([C@@H]([C@H](C(O1)O)O)F)O)O |
Source
|
Source | PubChem | |
URL | https://pubchem.ncbi.nlm.nih.gov | |
Description | Data deposited in or computed by PubChem | |
Molecular Formula |
C6H11FO5 |
Source
|
Source | PubChem | |
URL | https://pubchem.ncbi.nlm.nih.gov | |
Description | Data deposited in or computed by PubChem | |
Molecular Weight |
182.15 g/mol |
Source
|
Source | PubChem | |
URL | https://pubchem.ncbi.nlm.nih.gov | |
Description | Data deposited in or computed by PubChem | |
Disclaimer and Information on In-Vitro Research Products
Please be aware that all articles and product information presented on BenchChem are intended solely for informational purposes. The products available for purchase on BenchChem are specifically designed for in-vitro studies, which are conducted outside of living organisms. In-vitro studies, derived from the Latin term "in glass," involve experiments performed in controlled laboratory settings using cells or tissues. It is important to note that these products are not categorized as medicines or drugs, and they have not received approval from the FDA for the prevention, treatment, or cure of any medical condition, ailment, or disease. We must emphasize that any form of bodily introduction of these products into humans or animals is strictly prohibited by law. It is essential to adhere to these guidelines to ensure compliance with legal and ethical standards in research and experimentation.