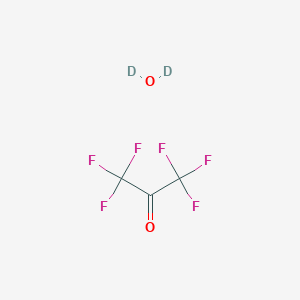
Deuterated water;1,1,1,3,3,3-hexafluoropropan-2-one
Overview
Description
Deuterated water;1,1,1,3,3,3-hexafluoropropan-2-one is a useful research compound. Its molecular formula is C3D2F6O2 and its molecular weight is 186.05. The purity is usually 95%.
BenchChem offers high-quality this compound suitable for many research applications. Different packaging options are available to accommodate customers' requirements. Please inquire for more information about this compound including the price, delivery time, and more detailed information at info@benchchem.com.
Mechanism of Action
Target of Action
Deuterated Water: Deuterated water, also known as heavy water, is a form of water where the hydrogen atoms are replaced by deuterium . The primary targets of deuterated water are the metabolic processes within the body . Deuterium, being a stable isotope of hydrogen, can replace hydrogen in many biochemical reactions, thereby affecting the metabolic stability of drugs .
1,1,1,3,3,3-Hexafluoropropan-2-one: 1,1,1,3,3,3-Hexafluoropropan-2-one is a highly polar solvent . It primarily targets chemical reactions, facilitating Friedel–Crafts-type reactions . It also enhances the efficiency of certain catalyzed reactions .
Mode of Action
Deuterated Water: Deuterated water interacts with its targets by replacing the hydrogen atoms in the metabolic reactions . This replacement leads to amplified bond strength, which in turn increases the biological half-life and thus metabolic stability of the drug .
1,1,1,3,3,3-Hexafluoropropan-2-one: 1,1,1,3,3,3-Hexafluoropropan-2-one interacts with its targets by facilitating reactions that would otherwise require a Lewis acid catalyst . It also enhances the efficiency of certain catalyzed reactions .
Biochemical Pathways
Deuterated Water: Deuterated water affects various biochemical pathways. It can result in metabolic shunting, leading to decreased exposure of critical organs to unwanted and toxic metabolites or increased exposure to desired active metabolites .
1,1,1,3,3,3-Hexafluoropropan-2-one: It is known to facilitate friedel–crafts-type reactions .
Pharmacokinetics
Deuterated Water: Deuterated water can demonstrate better pharmacokinetic or toxicological properties due to stronger deuterium–carbon bonds by altering their metabolism . This results in the increased drug half-life without affecting protein-binding interactions of the drug .
1,1,1,3,3,3-Hexafluoropropan-2-one:Result of Action
Deuterated Water: The molecular and cellular effects of deuterated water’s action include changes in fundamental processes such as cell division or energy metabolism . It can also lead to a decrease in the growth rate of mammalian cell lines .
1,1,1,3,3,3-Hexafluoropropan-2-one:Action Environment
Deuterated Water: Environmental factors can influence the action, efficacy, and stability of deuterated water. For example, the presence of heavy water in the environment can make growth a challenging task for some organisms .
Properties
IUPAC Name |
deuterated water;1,1,1,3,3,3-hexafluoropropan-2-one | |
---|---|---|
Source | PubChem | |
URL | https://pubchem.ncbi.nlm.nih.gov | |
Description | Data deposited in or computed by PubChem | |
InChI |
InChI=1S/C3F6O.H2O/c4-2(5,6)1(10)3(7,8)9;/h;1H2/i/hD2 | |
Source | PubChem | |
URL | https://pubchem.ncbi.nlm.nih.gov | |
Description | Data deposited in or computed by PubChem | |
InChI Key |
HEBNOKIGWWEWCN-ZSJDYOACSA-N | |
Source | PubChem | |
URL | https://pubchem.ncbi.nlm.nih.gov | |
Description | Data deposited in or computed by PubChem | |
Canonical SMILES |
C(=O)(C(F)(F)F)C(F)(F)F.O | |
Source | PubChem | |
URL | https://pubchem.ncbi.nlm.nih.gov | |
Description | Data deposited in or computed by PubChem | |
Isomeric SMILES |
[2H]O[2H].C(=O)(C(F)(F)F)C(F)(F)F | |
Source | PubChem | |
URL | https://pubchem.ncbi.nlm.nih.gov | |
Description | Data deposited in or computed by PubChem | |
Molecular Formula |
C3H2F6O2 | |
Source | PubChem | |
URL | https://pubchem.ncbi.nlm.nih.gov | |
Description | Data deposited in or computed by PubChem | |
DSSTOX Substance ID |
DTXSID90584208 | |
Record name | Hexafluoropropan-2-one--(~2~H_2_)water (1/1) | |
Source | EPA DSSTox | |
URL | https://comptox.epa.gov/dashboard/DTXSID90584208 | |
Description | DSSTox provides a high quality public chemistry resource for supporting improved predictive toxicology. | |
Molecular Weight |
186.05 g/mol | |
Source | PubChem | |
URL | https://pubchem.ncbi.nlm.nih.gov | |
Description | Data deposited in or computed by PubChem | |
CAS No. |
72301-81-6 | |
Record name | Hexafluoropropan-2-one--(~2~H_2_)water (1/1) | |
Source | EPA DSSTox | |
URL | https://comptox.epa.gov/dashboard/DTXSID90584208 | |
Description | DSSTox provides a high quality public chemistry resource for supporting improved predictive toxicology. | |
Record name | 72301-81-6 | |
Source | European Chemicals Agency (ECHA) | |
URL | https://echa.europa.eu/information-on-chemicals | |
Description | The European Chemicals Agency (ECHA) is an agency of the European Union which is the driving force among regulatory authorities in implementing the EU's groundbreaking chemicals legislation for the benefit of human health and the environment as well as for innovation and competitiveness. | |
Explanation | Use of the information, documents and data from the ECHA website is subject to the terms and conditions of this Legal Notice, and subject to other binding limitations provided for under applicable law, the information, documents and data made available on the ECHA website may be reproduced, distributed and/or used, totally or in part, for non-commercial purposes provided that ECHA is acknowledged as the source: "Source: European Chemicals Agency, http://echa.europa.eu/". Such acknowledgement must be included in each copy of the material. ECHA permits and encourages organisations and individuals to create links to the ECHA website under the following cumulative conditions: Links can only be made to webpages that provide a link to the Legal Notice page. | |
Synthesis routes and methods I
Procedure details
Synthesis routes and methods II
Procedure details
Synthesis routes and methods III
Procedure details
Disclaimer and Information on In-Vitro Research Products
Please be aware that all articles and product information presented on BenchChem are intended solely for informational purposes. The products available for purchase on BenchChem are specifically designed for in-vitro studies, which are conducted outside of living organisms. In-vitro studies, derived from the Latin term "in glass," involve experiments performed in controlled laboratory settings using cells or tissues. It is important to note that these products are not categorized as medicines or drugs, and they have not received approval from the FDA for the prevention, treatment, or cure of any medical condition, ailment, or disease. We must emphasize that any form of bodily introduction of these products into humans or animals is strictly prohibited by law. It is essential to adhere to these guidelines to ensure compliance with legal and ethical standards in research and experimentation.