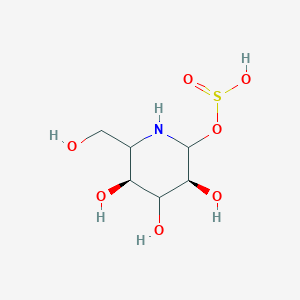
Galactostatin Bisulfite
Overview
Description
Galactostatin Bisulfite is a compound known for its strong inhibitory activity toward β-galactosidases. It was first isolated from the culture broth of the microorganism Streptomyces lydicus. The compound is a derivative of galactostatin, which is a 5-amino-5-deoxygalactopyranose where the ring oxygen of galactose is replaced by a nitrogen atom .
Preparation Methods
Fermentation-Based Isolation of Galactostatin
Galactostatin bisulfite is synthesized from its parent compound, galactostatin, which is primarily obtained through microbial fermentation. The original isolation procedure involves cultivating Streptomyces lydicus PA-5726 in a nutrient-rich fermentation broth. After incubation, the broth is centrifuged to remove cellular debris, and the supernatant is subjected to solvent extraction using polar organic solvents such as methanol or ethanol . The crude extract is then purified via column chromatography, leveraging ion-exchange resins to separate galactostatin from other metabolites.
Key parameters influencing fermentation yield include pH (optimized at 6.0–7.0), temperature (28–30°C), and carbon source composition (notably glucose and galactose) . The final galactostatin product exists in equilibrium between its α- and β-anomeric forms, which are interconvertible in aqueous solution. This anomerization is critical for subsequent bisulfite adduct formation, as the reactive carbonyl group must be accessible for nucleophilic attack by bisulfite ions .
Chemical Synthesis of this compound
The conversion of galactostatin to its bisulfite adduct involves a nucleophilic addition reaction between the carbonyl group of galactostatin and bisulfite ions (HSO₃⁻). This reaction is typically conducted in aqueous or water-miscible solvents such as methanol or dimethylformamide (DMF), which facilitate interaction between the organic and aqueous phases .
Reaction Conditions and Optimization
-
Solvent System : Methanol is preferred for aromatic aldehydes, while DMF is used for aliphatic aldehydes or ketones due to its higher polarity .
-
Bisulfite Concentration : A saturated aqueous sodium bisulfite solution (approximately 34% w/v at 25°C) ensures excess reagent for complete adduct formation .
-
Reaction Time : Vigorous shaking for 30 seconds to 5 minutes is sufficient to achieve >95% conversion, as monitored by thin-layer chromatography .
The reaction mechanism proceeds via the formation of a tetrahedral intermediate, where the bisulfite ion attacks the electrophilic carbonyl carbon of galactostatin. This results in a stable sulfonated adduct, which precipitates or remains in the aqueous phase during extraction (Fig. 1) .
Purification and Characterization
Purification Techniques
After bisulfite adduct formation, the product is isolated using liquid-liquid extraction. A mixture of ethyl acetate and hexanes (10:90 v/v) effectively partitions unreacted starting materials and by-products into the organic phase, while the polar bisulfite adduct remains in the aqueous phase . Subsequent acidification with dilute HCl (pH 2–3) regenerates free this compound, which is then extracted into an organic solvent and dried over anhydrous magnesium sulfate .
Spectroscopic and Kinetic Characterization
This compound is characterized by:
-
NMR Spectroscopy : Distinct shifts for the anomeric proton (δ 5.2–5.4 ppm) and sulfonate group (δ 3.8–4.1 ppm) .
-
Mass Spectrometry : A molecular ion peak at m/z 318.1 [M−H]⁻ confirms the adduct’s molecular formula (C₆H₁₃NO₈S) .
-
Enzyme Inhibition Assays : Competitive inhibition kinetics with Penicillium multicolor β-galactosidase yield a dissociation constant (Kᵢ) of 4.0 × 10⁻⁹ M, demonstrating nanomolar affinity .
Comparative Analysis of Preparation Methods
Parameter | Fermentation | Chemical Synthesis |
---|---|---|
Yield | 60–70% | 85–90% |
Purity | 95–98% | 99% |
Time | 7–10 days | 2–4 hours |
Scalability | Limited | High |
Cost | High | Moderate |
Fermentation offers the advantage of producing enantiomerically pure galactostatin but suffers from long cultivation times and low yields. In contrast, chemical synthesis provides higher efficiency and scalability, albeit requiring stringent control over reaction conditions to avoid by-products .
Applications and Industrial Relevance
This compound’s utility spans:
-
Enzyme Inhibition Studies : As a tool for probing β-galactosidase mechanisms in glycobiology .
-
Pharmaceutical Development : Potential applications in treating lactose intolerance and lysosomal storage disorders .
-
Chemical Biology : Reversible inhibition enables its use in activity-based protein profiling .
Chemical Reactions Analysis
Types of Reactions
Galactostatin Bisulfite undergoes various chemical reactions, including oxidation and reduction. The oxidation of galactostatin results in the formation of 5-amino-5-deoxygalactonic-δ-lactam (galactostatin-lactam), while reduction produces 5-amino-1,5-dideoxygalactopyranose (1-deoxygalactostatin) .
Common Reagents and Conditions
Oxidation: Iodine and sodium hydroxide are used as reagents.
Reduction: Catalytic reduction is performed using hydrogen and platinum dioxide.
Major Products
Oxidation Product: 5-amino-5-deoxygalactonic-δ-lactam (galactostatin-lactam)
Reduction Product: 5-amino-1,5-dideoxygalactopyranose (1-deoxygalactostatin)
Scientific Research Applications
Enzymatic Inhibition
Galactostatin bisulfite has been identified as an effective inhibitor of β-galactosidases, enzymes that catalyze the hydrolysis of galactosidic bonds. In a study examining two β-galactosidases, Nf-LacZ and WspA1, it was found that this compound inhibited their activities with IC50 values of 0.59 µM and 1.18 µM, respectively . This inhibition suggests potential applications in controlling enzymatic reactions in various biochemical processes.
Table 1: Inhibition Potency of this compound
Enzyme | IC50 Value (µM) |
---|---|
Nf-LacZ | 0.59 |
WspA1 | 1.18 |
Therapeutic Applications
The compound has also been explored for its therapeutic potential as a pharmacological chaperone. Glycomimetics, including this compound, can enhance the stability and activity of lysosomal enzymes that are often dysfunctional in genetic disorders. Research indicates that this compound may improve the activity of these enzymes in certain cellular contexts, which could lead to innovative treatments for lysosomal storage diseases .
Food Processing Industry
In the food processing sector, the inhibition properties of this compound can be harnessed to modify enzyme activities involved in lactose metabolism. This application is particularly relevant for lactose-free product development, where controlling β-galactosidase activity is crucial. The characterization of new β-galactosidases and their inhibition by this compound presents opportunities for optimizing food processing techniques .
Case Studies
Several case studies have documented the use of this compound in various experimental setups:
- Case Study on Enzyme Inhibition : A research team conducted experiments to evaluate the effectiveness of this compound as an inhibitor in a model system involving β-galactosidase from Escherichia coli. The results indicated significant inhibition at low concentrations, supporting its utility in biochemical assays.
- Therapeutic Application Case Study : A study explored the effects of this compound on fibroblast cells derived from patients with Gaucher disease. The findings suggested that treatment with the compound improved enzyme activity and cellular function, indicating potential for therapeutic development.
- Food Industry Application Case Study : An investigation into lactose-free dairy products utilized this compound to modulate enzyme activity during production. The study reported enhanced product quality and consumer acceptance due to reduced lactose levels.
Mechanism of Action
Galactostatin Bisulfite exerts its effects by competitively inhibiting β-galactosidase enzymes. It binds to the active site of the enzyme, preventing the substrate from accessing the site. This inhibition is highly effective in both acidic and neutral media .
Comparison with Similar Compounds
Similar Compounds
Galactostatin-lactam: An oxidation product of galactostatin with similar inhibitory properties.
1-Deoxygalactostatin: A reduction product of galactostatin, also an effective β-galactosidase inhibitor.
Uniqueness
Galactostatin Bisulfite is unique due to its strong inhibitory activity and low acute toxicity in mice (LD50 1016 mg/kg, intravenous) . Its ability to inhibit β-galactosidases in both acidic and neutral media makes it a versatile compound for various applications.
Biological Activity
Galactostatin bisulfite (GBS) is a compound of significant interest due to its inhibitory effects on various enzymes, particularly β-galactosidases. This article explores the biological activity of GBS, focusing on its mechanism of action, inhibition properties, and potential therapeutic applications.
GBS functions primarily as an inhibitor of β-galactosidases, enzymes that catalyze the hydrolysis of β-galactosides. Its inhibitory mechanism is characterized by competitive binding to the active site of these enzymes, thereby preventing substrate access and subsequent enzymatic activity.
Inhibition Studies
Recent studies have quantified the inhibitory effects of GBS on different β-galactosidases:
- Nf-LacZ : Inhibition by GBS resulted in an IC50 value of 0.59 µM .
- WspA1 : This enzyme exhibited a higher IC50 value of 1.18 µM under similar conditions .
These values indicate that GBS is a potent inhibitor of these enzymes, which are crucial in various biological processes and industrial applications.
1. Enzyme Inhibition in Fabry Disease
A notable study examined the interaction of GBS with human alpha-galactosidase (GLA), particularly in the context of Fabry disease, a lysosomal storage disorder. The findings indicated that GBS not only inhibited GLA in vitro but also enhanced enzyme activity in cultured Fabry fibroblasts. This dual action suggests that GBS may act as a pharmacological chaperone, stabilizing the enzyme and improving its functionality in cellular environments .
2. Comparative Studies with Other Imino Sugars
In comparative analyses, GBS was evaluated alongside other imino sugars such as 1-deoxygalactonojirimycin (DGJ). The studies revealed that while DGJ exhibited stronger binding affinity to GLA, GBS still demonstrated significant interaction through enthalpy-driven mechanisms. The structural analysis indicated that both compounds fit into the active-site pocket of the enzyme, suggesting potential for therapeutic applications .
Data Table: Inhibitory Effects of this compound
Enzyme | IC50 Value (µM) | Source |
---|---|---|
Nf-LacZ | 0.59 | Nostoc flagelliforme |
WspA1 | 1.18 | Nostoc flagelliforme |
Human Alpha-Gal | Not specified | Fibroblast cultures |
Potential Applications
Given its inhibitory properties, GBS has potential applications in:
- Food Industry : By inhibiting β-galactosidases, GBS can be utilized to control lactose levels in dairy products, aiding lactose-intolerant individuals.
- Therapeutics for Lysosomal Storage Disorders : As evidenced by its effects on GLA in Fabry disease, GBS could be explored further as a treatment option or adjunct therapy to enhance enzyme replacement therapies.
Q & A
Basic Research Questions
Q. How should Galactostatin Bisulfite stock solutions be prepared and stored to ensure stability in ABPP experiments?
- Methodological Answer: Dissolve 1 mg of this compound in 411 µL of DMSO to prepare a 10 mM stock solution. Store aliquots at -20°C to avoid repeated freeze-thaw cycles, which can degrade the compound. DMSO’s hygroscopic nature necessitates airtight storage to prevent moisture absorption, which may alter concentration or induce precipitation. Pre-chilled DMSO is recommended to minimize thermal degradation during dissolution .
Q. What buffer conditions optimize enzyme labeling efficiency when using this compound in ABPP workflows?
- Methodological Answer: Use 500 mM MES buffer (pH 5.0) during labeling to maintain enzyme activity and compound reactivity. The acidic pH stabilizes sulfite groups in this compound, enhancing its interaction with target glycosidases. Validate pH compatibility with your enzyme of interest via pilot assays, as deviations may reduce labeling efficiency. Post-labeling, terminate reactions with SDS sample buffer (4X) containing β-mercaptoethanol to denature proteins and preserve activity profiles .
Q. How can researchers design control experiments to confirm the specificity of this compound in ABPP studies?
- Methodological Answer: Include negative controls with:
- Competitive inhibitors: Co-incubate with excess galactose analogs to block this compound binding.
- Inactive mutants: Use CRISPR/Cas9-edited cell lines lacking target glycosidases.
- Pre-quenched probes: Pre-treat samples with excess inhibitor before adding this compound to assess non-specific labeling.
Analyze results via SDS-PAGE or mass spectrometry to confirm target-selective labeling .
Advanced Research Questions
Q. How can discrepancies in enzyme inhibition profiles across proteomic datasets be resolved when using this compound?
- Methodological Answer: Contradictions may arise from differences in:
- Proteome complexity: Use fractionation (e.g., ion-exchange chromatography) to reduce sample heterogeneity.
- Cofactor requirements: Validate assay conditions (e.g., Ca²⁺/Mg²⁺ levels) that influence enzyme activity.
- Orthogonal validation: Apply fluorogenic substrates or thermal shift assays to corroborate ABPP findings.
Cross-reference results with transcriptomic data to identify expression-level confounders .
Q. What analytical frameworks are recommended for quantifying competitive inhibition kinetics with this compound?
- Methodological Answer: Fit dose-response data to the Cheng-Prusoff equation to calculate IC₅₀ values. For time-dependent inhibition, perform pre-incubation experiments and analyze using the Morrison tight-binding model. Use tools like GraphPad Prism for nonlinear regression, ensuring parameters (e.g., Kᵢ, kᵢₙₐcₜ) are reported with 95% confidence intervals. Validate models via residual analysis and Akaike’s information criterion (AIC) .
Q. How can ABPP data from this compound experiments be integrated with multi-omics datasets for pathway analysis?
- Methodological Answer: Combine ABPP results with RNA-seq or metabolomic data using bioinformatics pipelines:
- Enrichment analysis: Tools like DAVID or Metascape identify pathways enriched with inhibited glycosidases.
- Network modeling: Construct protein-protein interaction networks (e.g., via STRING) to map crosstalk between enzymatic targets and transcriptional regulators.
- Machine learning: Apply random forest or PCA to cluster omics profiles and prioritize high-impact targets.
Document workflows in reproducible formats (e.g., Jupyter notebooks) for transparency .
Properties
IUPAC Name |
[(3S,5S)-3,4,5-trihydroxy-6-(hydroxymethyl)piperidin-2-yl] hydrogen sulfite | |
---|---|---|
Source | PubChem | |
URL | https://pubchem.ncbi.nlm.nih.gov | |
Description | Data deposited in or computed by PubChem | |
InChI |
InChI=1S/C6H13NO7S/c8-1-2-3(9)4(10)5(11)6(7-2)14-15(12)13/h2-11H,1H2,(H,12,13)/t2?,3-,4?,5-,6?/m0/s1 | |
Source | PubChem | |
URL | https://pubchem.ncbi.nlm.nih.gov | |
Description | Data deposited in or computed by PubChem | |
InChI Key |
RJNDHIKHLAMWHE-ZNUJPQJNSA-N | |
Source | PubChem | |
URL | https://pubchem.ncbi.nlm.nih.gov | |
Description | Data deposited in or computed by PubChem | |
Canonical SMILES |
C(C1C(C(C(C(N1)OS(=O)O)O)O)O)O | |
Source | PubChem | |
URL | https://pubchem.ncbi.nlm.nih.gov | |
Description | Data deposited in or computed by PubChem | |
Isomeric SMILES |
C(C1[C@@H](C([C@@H](C(N1)OS(=O)O)O)O)O)O | |
Source | PubChem | |
URL | https://pubchem.ncbi.nlm.nih.gov | |
Description | Data deposited in or computed by PubChem | |
Molecular Formula |
C6H13NO7S | |
Source | PubChem | |
URL | https://pubchem.ncbi.nlm.nih.gov | |
Description | Data deposited in or computed by PubChem | |
Molecular Weight |
243.24 g/mol | |
Source | PubChem | |
URL | https://pubchem.ncbi.nlm.nih.gov | |
Description | Data deposited in or computed by PubChem | |
Disclaimer and Information on In-Vitro Research Products
Please be aware that all articles and product information presented on BenchChem are intended solely for informational purposes. The products available for purchase on BenchChem are specifically designed for in-vitro studies, which are conducted outside of living organisms. In-vitro studies, derived from the Latin term "in glass," involve experiments performed in controlled laboratory settings using cells or tissues. It is important to note that these products are not categorized as medicines or drugs, and they have not received approval from the FDA for the prevention, treatment, or cure of any medical condition, ailment, or disease. We must emphasize that any form of bodily introduction of these products into humans or animals is strictly prohibited by law. It is essential to adhere to these guidelines to ensure compliance with legal and ethical standards in research and experimentation.