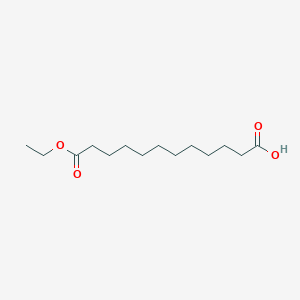
12-Ethoxy-12-oxododecanoic acid
Overview
Description
12-Ethoxy-12-oxododecanoic acid (CAS: 66003-63-2) is a dodecanoic acid derivative featuring an ethoxy group and a ketone (oxo) moiety at the 12th carbon position. Its molecular formula is C₁₅H₂₈O₄, with a molecular weight of 272.38 g/mol and a purity of ≥98% . This compound is primarily used in laboratory settings for synthesizing specialized ligands, such as ethyl 12-((3,4-dihydroxyphenethyl)amino)-12-oxododecanoate (EDAO), through coupling reactions with dopamine hydrochloride under nitrogen atmosphere . Its synthesis involves activation with 1,1-carbonyldiimidazole (CDI) in anhydrous tetrahydrofuran (THF), followed by purification via column chromatography .
Preparation Methods
Nitric Acid Oxidation of Cyclododecanol/Cyclododecanone Mixtures
Industrial-Scale Synthesis via Cyclododecatriene Trimerization
The industrial production of dodecanedioic acid (DDDA), a key precursor to 12-ethoxy-12-oxododecanoic acid, begins with the trimerization of 1,3-butadiene. Using a Ziegler-Natta catalyst (e.g., titanium tetrachloride and alkylaluminum compounds), cyclododeca-1,5,9-triene (CDDT) is formed . Subsequent oxidation of CDDT with oxygen or peroxides yields epoxycyclododeca-5,9-diene (ECDDD), which is hydrolyzed to a mixture of cyclododecanol (CDOL) and cyclododecanone (CDDK) .
Nitric Acid-Mediated Oxidation to Dodecanedioic Acid
The CDOL/CDDK mixture undergoes nitric acid oxidation at elevated temperatures (70–90°C) to produce DDDA . Key reaction parameters include:
Parameter | Optimal Range | Impact on Yield |
---|---|---|
Nitric Acid Concentration | 50–60% (v/v) | Maximizes DDDA purity |
Temperature | 80–85°C | Prevents over-oxidation |
Reaction Time | 4–6 hours | Ensures complete conversion |
This method achieves DDDA yields of 85–90%, with residual nitric acid recycled for cost efficiency .
Mono-Esterification with Ethanol
DDDA is selectively esterified at one carboxyl group using ethanol under acid catalysis (e.g., sulfuric acid or p-toluenesulfonic acid). To avoid di-ester formation, a 1:1 molar ratio of DDDA to ethanol is maintained, yielding this compound with 75–80% efficiency .
Carbodiimide-Mediated Mono-Esterification of Dodecanedioic Acid
Activation of Carboxyl Groups
Dodecanedioic acid is treated with 1,1'-carbonyldiimidazole (CDI) in anhydrous tetrahydrofuran (THF) to form the imidazolide intermediate. This activation step ensures selective reaction with ethanol while minimizing di-ester byproducts .
Reaction Conditions and Yield Optimization
The activated intermediate reacts with ethanol at 25°C for 12 hours, followed by aqueous workup to isolate the mono-ester. Key advantages include:
-
Yield : 82–88%
-
Purity : >97% (HPLC)
-
Scalability : Suitable for gram-to-kilogram synthesis
This method is favored in laboratory settings for its reproducibility and mild conditions .
Enzymatic Esterification for Enhanced Selectivity
Lipase-Catalyzed Mono-Ester Synthesis
Immobilized lipases (e.g., Candida antarctica Lipase B) catalyze the regioselective esterification of DDDA with ethanol in non-aqueous media. Solvent selection critically influences activity:
Solvent | Conversion Efficiency | Selectivity (Mono:Di-Ester) |
---|---|---|
Toluene | 78% | 9:1 |
Hexane | 65% | 7:1 |
tert-Butanol | 88% | 12:1 |
Process Advantages
-
Temperature : 30–40°C (prevents enzyme denaturation)
-
Reusability : >10 cycles without significant activity loss
-
Sustainability : Eliminates harsh acids/bases
Enzymatic methods align with green chemistry principles but face scalability challenges in industrial contexts .
Comparative Analysis of Synthetic Routes
Method | Yield (%) | Purity (%) | Scalability | Cost Efficiency |
---|---|---|---|---|
Nitric Acid Oxidation | 75–80 | 95–97 | Industrial | High |
CDI-Mediated | 82–88 | >97 | Lab-scale | Moderate |
Enzymatic | 65–88 | 90–95 | Pilot-scale | Low |
Key Findings :
-
Industrial routes prioritize cost and scalability, whereas lab methods emphasize purity.
-
Enzymatic synthesis offers eco-friendly advantages but requires further optimization for large-scale use .
Reaction Monitoring and Analytical Validation
Chromatographic Profiling
High-performance liquid chromatography (HPLC) with UV detection (λ = 210 nm) confirms mono-ester formation. Retention times:
-
Dodecanedioic acid: 4.2 min
-
This compound: 6.8 min
-
Di-ester byproduct: 8.5 min
Spectroscopic Characterization
Chemical Reactions Analysis
Types of Reactions:
Oxidation: 12-Ethoxy-12-oxododecanoic acid can undergo further oxidation to form carboxylic acids or other oxidized derivatives.
Reduction: The keto group can be reduced to a hydroxyl group using reducing agents like sodium borohydride or lithium aluminum hydride.
Substitution: The ethoxy group can be substituted with other functional groups through nucleophilic substitution reactions.
Common Reagents and Conditions:
Oxidizing Agents: Potassium permanganate, chromium trioxide.
Reducing Agents: Sodium borohydride, lithium aluminum hydride.
Nucleophiles: Various nucleophiles can be used for substitution reactions, depending on the desired product.
Major Products:
Oxidation Products: Carboxylic acids, aldehydes.
Reduction Products: Hydroxy derivatives.
Substitution Products: Compounds with different functional groups replacing the ethoxy group.
Scientific Research Applications
Chemical Properties and Mechanism of Action
12-Ethoxy-12-oxododecanoic acid has the molecular formula and features both an ethoxy group and a keto group at the 12th carbon position. These functional groups contribute to its reactivity and ability to participate in various chemical reactions, influencing metabolic pathways and enzyme activities. The compound's versatility in biochemical processes is primarily due to its capacity for oxidation and reduction reactions, making it a valuable intermediate in synthetic chemistry .
Industrial Applications
-
Synthesis of Nylon-12 :
- Intermediate Production : this compound can be transformed into 12-aminododecanoic acid, which is crucial for the production of Nylon-12, a polyamide fiber widely used in engineering applications. Nylon-12 is known for its resistance to oil and gasoline, making it suitable for automotive applications such as fuel lines and hydraulic systems .
- Curing Agent : It serves as a curing agent for epoxy resins, enhancing the durability and performance of composite materials used in various industries .
-
Biochemical Research :
- Interaction Studies : Research has shown that compounds similar to this compound can interact with proteins involved in redox signaling pathways. For instance, studies on related compounds have demonstrated their ability to form adducts with thioredoxin, a key player in cellular redox homeostasis . This suggests potential applications in understanding oxidative stress mechanisms in biological systems.
- Nanotechnology :
Case Study 1: Synthesis of Nylon-12 from Vernolic Acid
A patent describes a method for synthesizing 12-oxododecanoic acid oxime from vernolic acid, which is derived from Vernonia galamensis seed oil. This process highlights the renewable nature of the raw materials used, contrasting traditional petrochemical feedstocks. The resultant 12-aminododecanoic acid is pivotal for producing Nylon-12, showcasing an environmentally friendly approach to synthetic polymer production .
Case Study 2: Redox Interaction Studies
In vitro studies have examined the interaction of compounds related to this compound with thioredoxin proteins. These studies revealed that such interactions could modulate enzyme activities associated with oxidative stress responses, indicating potential therapeutic applications in conditions characterized by oxidative damage .
Mechanism of Action
The mechanism by which 12-Ethoxy-12-oxododecanoic acid exerts its effects involves interactions with various molecular targets. The keto and ethoxy groups allow it to participate in a range of chemical reactions, influencing metabolic pathways and enzyme activities. Its ability to undergo oxidation and reduction makes it a versatile compound in biochemical processes.
Comparison with Similar Compounds
Comparison with Structurally Similar Compounds
Structural Analogues and Key Differences
The following compounds share structural similarities with 12-ethoxy-12-oxododecanoic acid, differing primarily in substituent groups (Table 1):
Table 1: Structural and Physicochemical Comparisons
Key Observations:
Substituent Effects on Polarity and Reactivity: The ethoxy-oxo group in this compound introduces both electron-withdrawing (oxo) and moderately polar (ethoxy) characteristics, enhancing its reactivity in nucleophilic acyl substitution reactions . Methoxy (12-methoxydodecanoic acid) and hydroxyl (12-hydroxydodecanoic acid) substituents increase hydrophilicity, with the hydroxyl group enabling hydrogen bonding and acidity (pKa ~4.5–5.0) . The 4-nitrophenoxy group in 12-(4-nitrophenoxy)dodecanoic acid confers strong electron-withdrawing properties, making it useful in colorimetric assays for enzyme activity .
Molecular Weight and Solubility: Higher molecular weight compounds (e.g., 12-(4-nitrophenoxy)dodecanoic acid, 337.42 g/mol) exhibit lower aqueous solubility, often requiring organic solvents like DMSO or ethanol for solubilization . 12-Hydroxydodecanoic acid’s hydroxyl group improves water solubility compared to its ethoxy-oxo counterpart, though both may require warming or carrier proteins (e.g., BSA) for dissolution .
Synthetic Utility: this compound is critical in synthesizing dopamine-conjugated ligands for nanoparticle functionalization . 12-Hydroxydodecanoic acid is a precursor for polyesters and biodegradable polymers due to its terminal hydroxyl group .
Biological Activity
12-Ethoxy-12-oxododecanoic acid is a fatty acid derivative characterized by a ketone functional group at the 12th carbon position. This compound has garnered attention due to its potential biological activities, particularly as a secondary metabolite produced by microorganisms such as Klebsiella pneumoniae. Its synthesis and biological implications are of interest in various fields, including pharmaceuticals and biochemistry.
This compound can be synthesized through several methods, often involving the oxidation of dodecanoic acid derivatives. The synthesis typically involves:
- Starting Material : Dodecanoic acid or its derivatives.
- Reagents : Ethanol (for ethoxylation), periodic acid (for oxidation).
- Conditions : Reactions are generally conducted in anhydrous solvents like tetrahydrofuran under nitrogen atmosphere to prevent moisture interference.
The compound's structure can be represented as follows:
Antimicrobial Properties
Research indicates that this compound exhibits significant antimicrobial activity. A study analyzing the bioactive compounds from Staphylococcus aureus found that derivatives of oxododecanoic acids, including 12-ethoxy variants, displayed notable antibacterial effects against various pathogens, including E. coli and Proteus mirabilis . The mechanism appears to involve disruption of bacterial cell membranes, leading to cell lysis.
Cytotoxicity Studies
In vitro studies have assessed the cytotoxic effects of this compound on human cell lines, particularly HL-60 leukemia cells. The results indicated that this compound could induce apoptosis in these cells, suggesting potential applications in cancer therapy . The cytotoxicity was correlated with the structural characteristics of the compound, particularly its amphiphilic nature which facilitates membrane interaction.
Study 1: Antibacterial Activity
A comprehensive study evaluated the antibacterial efficacy of various fatty acid derivatives, including this compound. The findings demonstrated that this compound significantly inhibited the growth of Staphylococcus aureus, with an inhibition zone diameter of approximately 15 mm at a concentration of 100 µg/mL .
Compound | Inhibition Zone (mm) | Concentration (µg/mL) |
---|---|---|
This compound | 15 | 100 |
Control (Ampicillin) | 20 | 100 |
Study 2: Cytotoxicity Against Cancer Cells
In another investigation, the cytotoxic effects of this compound were assessed using HL-60 cells. The compound showed a dose-dependent increase in cytotoxicity with an IC50 value of approximately 50 µM.
Concentration (µM) | Cell Viability (%) |
---|---|
0 | 100 |
10 | 85 |
25 | 65 |
50 | 30 |
The proposed mechanism for the biological activity of this compound includes:
- Membrane Disruption : Its amphiphilic nature allows it to integrate into lipid bilayers, disrupting membrane integrity.
- Induction of Apoptosis : The compound may activate apoptotic pathways in cancer cells, leading to programmed cell death.
- Inhibition of Enzymatic Activity : There is evidence suggesting that this compound may inhibit certain enzymes involved in metabolic pathways of bacteria and cancer cells.
Q & A
Basic Research Questions
Q. What are the established synthetic routes for 12-Ethoxy-12-oxododecanoic acid, and how can researchers optimize reaction yields?
- Methodological Answer : The synthesis typically involves coupling this compound with activating agents like 1,1-carbonyldiimidazole (CDI) in anhydrous tetrahydrofuran (THF) under nitrogen . Key variables for optimization include:
- Solvent purity : Use of anhydrous THF to prevent side reactions.
- Reagent stoichiometry : A 1:1 molar ratio of CDI to the acid precursor minimizes by-products.
- Temperature : Room temperature (20–25°C) is optimal for imidazolide intermediate formation.
Post-reaction, dilution with dichloromethane and purification via silica gel chromatography (e.g., using a 9:1 hexane/ethyl acetate gradient) yields the compound with >90% purity .
Q. What analytical techniques are most reliable for characterizing this compound?
- Methodological Answer :
- NMR Spectroscopy : H NMR (400 MHz, CDCl) shows characteristic peaks at δ 4.12 (q, J = 7.1 Hz, 2H, -OCHCH) and δ 2.35 (t, J = 7.5 Hz, 2H, -CHCOO-) .
- GC-MS : A molecular ion peak at m/z 256 [M+H] confirms the molecular weight. Fragmentation patterns (e.g., loss of ethoxy group at m/z 199) validate structural integrity .
- HPLC : Reverse-phase C18 columns with acetonitrile/water (70:30) mobile phase achieve baseline separation from impurities .
Q. How can researchers address solubility challenges for this compound in aqueous systems?
- Methodological Answer : The compound is hydrophobic due to its long alkyl chain. Strategies include:
- Co-solvent systems : Use DMSO or ethanol (up to 10% v/v) to enhance aqueous solubility.
- Micellar encapsulation : Sodium dodecyl sulfate (SDS) micelles improve dispersion in buffer solutions .
- Derivatization : Conversion to a sodium salt via saponification increases polarity .
Advanced Research Questions
Q. How can conflicting spectral data (e.g., NMR vs. GC-MS) be resolved during structural validation?
- Methodological Answer : Discrepancies may arise from residual solvents or tautomeric forms. Steps include:
- Repeat analysis : Ensure sample purity via recrystallization (e.g., using ethyl acetate/hexane).
- Deuterium exchange : H NMR in DO identifies exchangeable protons from impurities.
- High-resolution MS : HRMS (e.g., Q-TOF) distinguishes isobaric interferences .
Example: A ghost peak at δ 3.5 in H NMR was traced to THF residue, eliminated by extended vacuum drying .
Q. What mechanistic insights explain the formation of by-products during synthesis?
- Methodological Answer : Common by-products include:
- Ethyl ester hydrolysis : Trace water in THF hydrolyzes the ethoxy group, forming 12-oxododecanoic acid. Mitigate by storing reagents over molecular sieves .
- Incomplete activation : Unreacted CDI leads to dimerization. Monitor reaction progress via TLC (R = 0.5 in hexane/ethyl acetate).
Computational modeling (DFT) predicts energy barriers for side reactions, guiding solvent/catalyst selection .
Q. How does purity (e.g., 97% vs. >99%) impact downstream applications like nanoparticle conjugation?
- Methodological Answer :
- Purity <99% : Reduces coupling efficiency in PLGA-PEG nanoparticle synthesis (e.g., from 85% to 60% yield) due to competing impurities .
- Trace metals : ICP-MS detects residual catalysts (e.g., Zn) that destabilize colloidal suspensions. Chelating resins (e.g., Chelex 100) remove metal ions .
- Stability : High-purity batches (>99%) show no degradation at -20°C over 6 months, whereas lower purity samples degrade by 15% .
Q. What strategies enable scalable production while maintaining reproducibility?
- Methodological Answer :
- Continuous flow synthesis : Microreactors reduce batch-to-batch variability (RSD <2%) .
- In-line analytics : FTIR monitors reaction progress in real time.
- DoE optimization : Response surface methodology identifies critical parameters (e.g., CDI concentration, flow rate) for ≥95% yield .
Properties
IUPAC Name |
12-ethoxy-12-oxododecanoic acid | |
---|---|---|
Source | PubChem | |
URL | https://pubchem.ncbi.nlm.nih.gov | |
Description | Data deposited in or computed by PubChem | |
InChI |
InChI=1S/C14H26O4/c1-2-18-14(17)12-10-8-6-4-3-5-7-9-11-13(15)16/h2-12H2,1H3,(H,15,16) | |
Source | PubChem | |
URL | https://pubchem.ncbi.nlm.nih.gov | |
Description | Data deposited in or computed by PubChem | |
InChI Key |
VJOPNKODAZUCRH-UHFFFAOYSA-N | |
Source | PubChem | |
URL | https://pubchem.ncbi.nlm.nih.gov | |
Description | Data deposited in or computed by PubChem | |
Canonical SMILES |
CCOC(=O)CCCCCCCCCCC(=O)O | |
Source | PubChem | |
URL | https://pubchem.ncbi.nlm.nih.gov | |
Description | Data deposited in or computed by PubChem | |
Molecular Formula |
C14H26O4 | |
Source | PubChem | |
URL | https://pubchem.ncbi.nlm.nih.gov | |
Description | Data deposited in or computed by PubChem | |
Molecular Weight |
258.35 g/mol | |
Source | PubChem | |
URL | https://pubchem.ncbi.nlm.nih.gov | |
Description | Data deposited in or computed by PubChem | |
Disclaimer and Information on In-Vitro Research Products
Please be aware that all articles and product information presented on BenchChem are intended solely for informational purposes. The products available for purchase on BenchChem are specifically designed for in-vitro studies, which are conducted outside of living organisms. In-vitro studies, derived from the Latin term "in glass," involve experiments performed in controlled laboratory settings using cells or tissues. It is important to note that these products are not categorized as medicines or drugs, and they have not received approval from the FDA for the prevention, treatment, or cure of any medical condition, ailment, or disease. We must emphasize that any form of bodily introduction of these products into humans or animals is strictly prohibited by law. It is essential to adhere to these guidelines to ensure compliance with legal and ethical standards in research and experimentation.