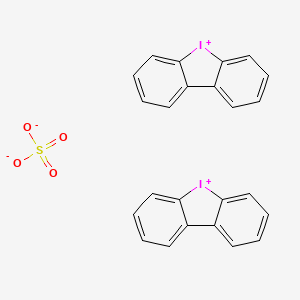
Diphenyleneiodonium sulfate
Overview
Description
Diphenyleneiodonium sulfate is a chemical compound known for its role as an inhibitor of NADPH oxidase. It is widely used in scientific research due to its ability to inhibit various flavoenzymes, including nitric oxide synthase and NADPH oxidase. This compound has significant applications in the fields of chemistry, biology, and medicine, particularly in studies related to oxidative stress and inflammation .
Mechanism of Action
Target of Action
Diphenyleneiodonium sulfate primarily targets NADPH oxidase 2 (NOX2) . NADPH oxidase is an enzyme that plays a crucial role in the production of reactive oxygen species (ROS), which are involved in various cellular processes, including cell signaling, immune response, and apoptosis .
Mode of Action
As an inhibitor of NADPH oxidase, this compound prevents the enzyme from producing ROS . This interaction results in a decrease in ROS levels within the cell, which can lead to changes in various cellular processes that are regulated by these molecules .
Biochemical Pathways
The inhibition of NADPH oxidase by this compound affects several biochemical pathways. For instance, it has been shown to regulate fatty acid and astaxanthin accumulation in certain organisms under stress conditions . Moreover, it can influence the metabolic mechanisms underlying fatty acid and carotenoid accumulation under abiotic stresses .
Pharmacokinetics
It is known that the compound can accumulate in the liver .
Result of Action
The action of this compound leads to various molecular and cellular effects. For example, it has been shown to lower whole body and blood glucose concentrations in rats . Additionally, it can induce a viable but non-culturable state in certain bacteria, similar to dormant bacilli .
Action Environment
The action, efficacy, and stability of this compound can be influenced by various environmental factors. For instance, its ability to inhibit NADPH oxidase and reduce ROS production can be particularly beneficial in environments where oxidative stress is high . .
Biochemical Analysis
Biochemical Properties
Diphenyleneiodonium sulfate plays a significant role in biochemical reactions by inhibiting enzymes such as NADPH oxidase, nitric oxide synthase, and xanthine oxidase . It interacts with these enzymes by binding to their flavin cofactors, thereby preventing the transfer of electrons and inhibiting their activity . This inhibition leads to a reduction in the production of reactive oxygen species (ROS), which are involved in various cellular processes and signaling pathways .
Cellular Effects
This compound has profound effects on various types of cells and cellular processes. It influences cell function by inhibiting the production of ROS, which are crucial for cell signaling pathways, gene expression, and cellular metabolism . For example, in lung epithelial cells and phagocytes, this compound reduces oxidative stress by inhibiting NADPH oxidase activity, leading to decreased protein and lipid oxidation . Additionally, it has been shown to activate the human TRPA1 nociceptor in human embryonic kidney cells and fibroblast-like synoviocytes, indicating its role in pain signaling .
Molecular Mechanism
The molecular mechanism of this compound involves its interaction with flavoenzymes, where it acts as an uncompetitive inhibitor . By binding to the flavin cofactors of these enzymes, this compound prevents the transfer of electrons, thereby inhibiting enzyme activity . This inhibition leads to a decrease in ROS production, which in turn affects various cellular processes such as inflammation and oxidative stress . Additionally, this compound has been shown to enhance P2X7-dependent non-opsonized phagocytosis and suppress inflammasome activation by blocking CX43-mediated ATP leakage .
Temporal Effects in Laboratory Settings
In laboratory settings, the effects of this compound can change over time. Studies have shown that it can prevent early alcohol-induced liver injury in rats by inhibiting NADPH oxidase activity and reducing oxidative stress . The compound’s stability and degradation over time can influence its long-term effects on cellular function. For example, in Parkinson’s disease models, post-treatment with ultra-low doses of this compound significantly attenuated disease progression and improved motor function .
Dosage Effects in Animal Models
The effects of this compound vary with different dosages in animal models. At ultra-low doses, it has been shown to reduce microglia-mediated chronic neuroinflammation and halt the progression of neurodegeneration in mouse models of Parkinson’s disease . Higher doses can lead to toxic effects, such as extreme and irreversible hypoglycemia in several animal species . Therefore, it is crucial to determine the appropriate dosage to achieve the desired therapeutic effects while minimizing adverse effects.
Metabolic Pathways
This compound is involved in various metabolic pathways, including the pentose phosphate pathway and the tricarboxylic acid cycle . It inhibits several NAD(P)-dependent enzymes, such as glucose 6-phosphate dehydrogenase, glyceraldehyde 3-phosphate dehydrogenase, and lactate dehydrogenase . This inhibition leads to a decrease in the production of NADPH, a crucial cofactor for many antioxidant enzymes, thereby affecting the overall redox balance within cells .
Transport and Distribution
Within cells and tissues, this compound is transported and distributed through various mechanisms. It has been shown to activate the efflux of radioiodide in thyroid cells, indicating its role as an iodide-specific transporter . Additionally, it is extensively accumulated by the liver and tightly bound by mitochondria . This accumulation can influence its localization and activity within different cellular compartments.
Subcellular Localization
The subcellular localization of this compound is crucial for its activity and function. It has been observed to accumulate in the plasma membrane, rough endoplasmic reticulum, and mitochondria of cells . This localization is essential for its role in inhibiting flavoenzymes and reducing oxidative stress within specific cellular compartments. Additionally, the compound’s interaction with various transporters and binding proteins can affect its distribution and localization within cells .
Preparation Methods
Synthetic Routes and Reaction Conditions
Diphenyleneiodonium sulfate can be synthesized through the reaction of diphenyleneiodonium chloride with sulfuric acid. The reaction typically involves the following steps:
- Dissolution of diphenyleneiodonium chloride in an appropriate solvent.
- Addition of sulfuric acid to the solution.
- Stirring the mixture at a controlled temperature to facilitate the reaction.
- Isolation and purification of the resulting this compound by filtration and recrystallization .
Industrial Production Methods
Industrial production methods for this compound are similar to laboratory synthesis but are scaled up to accommodate larger quantities. The process involves the use of industrial-grade solvents and reagents, along with optimized reaction conditions to ensure high yield and purity. The final product is subjected to rigorous quality control measures to meet industry standards .
Chemical Reactions Analysis
Types of Reactions
Diphenyleneiodonium sulfate undergoes various chemical reactions, including:
Oxidation: It acts as an inhibitor of NADPH oxidase, preventing the oxidation of NADPH to NADP+.
Reduction: It can be reduced under specific conditions, although this is less common.
Substitution: It can participate in substitution reactions, particularly in the presence of nucleophiles.
Common Reagents and Conditions
Oxidation: Common reagents include NADPH and oxygen, with the reaction typically occurring in aqueous solutions at physiological pH.
Reduction: Reducing agents such as sodium borohydride can be used under controlled conditions.
Substitution: Nucleophiles such as amines or thiols can react with this compound in organic solvents.
Major Products Formed
The major products formed from these reactions depend on the specific conditions and reagents used. For example, the inhibition of NADPH oxidase results in the prevention of reactive oxygen species (ROS) formation .
Scientific Research Applications
Diphenyleneiodonium sulfate has a wide range of scientific research applications:
Chemistry: It is used as a reagent to study the inhibition of flavoenzymes and to investigate redox reactions.
Biology: It is employed in studies of oxidative stress, inflammation, and cellular respiration.
Medicine: It has potential therapeutic applications in conditions related to oxidative stress, such as cancer and inflammatory diseases.
Industry: It is used in the development of new drugs and in the study of enzyme inhibition mechanisms .
Comparison with Similar Compounds
Similar Compounds
Apocynin: Another NADPH oxidase inhibitor with similar applications in oxidative stress research.
DPI (Diphenyleneiodonium chloride): A closely related compound with similar inhibitory effects on flavoenzymes
Uniqueness
Diphenyleneiodonium sulfate is unique due to its high potency and specificity as an NADPH oxidase inhibitor. It has a broader range of applications compared to other similar compounds, making it a valuable tool in scientific research .
Properties
IUPAC Name |
8-iodoniatricyclo[7.4.0.02,7]trideca-1(13),2,4,6,9,11-hexaene;sulfate | |
---|---|---|
Source | PubChem | |
URL | https://pubchem.ncbi.nlm.nih.gov | |
Description | Data deposited in or computed by PubChem | |
InChI |
InChI=1S/2C12H8I.H2O4S/c2*1-3-7-11-9(5-1)10-6-2-4-8-12(10)13-11;1-5(2,3)4/h2*1-8H;(H2,1,2,3,4)/q2*+1;/p-2 | |
Source | PubChem | |
URL | https://pubchem.ncbi.nlm.nih.gov | |
Description | Data deposited in or computed by PubChem | |
InChI Key |
PLJRZMICUGKBGN-UHFFFAOYSA-L | |
Source | PubChem | |
URL | https://pubchem.ncbi.nlm.nih.gov | |
Description | Data deposited in or computed by PubChem | |
Canonical SMILES |
C1=CC=C2C(=C1)C3=CC=CC=C3[I+]2.C1=CC=C2C(=C1)C3=CC=CC=C3[I+]2.[O-]S(=O)(=O)[O-] | |
Source | PubChem | |
URL | https://pubchem.ncbi.nlm.nih.gov | |
Description | Data deposited in or computed by PubChem | |
Molecular Formula |
C24H16I2O4S | |
Source | PubChem | |
URL | https://pubchem.ncbi.nlm.nih.gov | |
Description | Data deposited in or computed by PubChem | |
Related CAS |
244-54-2 (Parent) | |
Record name | Bis(dibenziodonium) sulphate | |
Source | ChemIDplus | |
URL | https://pubchem.ncbi.nlm.nih.gov/substance/?source=chemidplus&sourceid=0004510832 | |
Description | ChemIDplus is a free, web search system that provides access to the structure and nomenclature authority files used for the identification of chemical substances cited in National Library of Medicine (NLM) databases, including the TOXNET system. | |
DSSTOX Substance ID |
DTXSID701044278 | |
Record name | Dibenziodolium, sulfate (2:1) | |
Source | EPA DSSTox | |
URL | https://comptox.epa.gov/dashboard/DTXSID701044278 | |
Description | DSSTox provides a high quality public chemistry resource for supporting improved predictive toxicology. | |
Molecular Weight |
654.3 g/mol | |
Source | PubChem | |
URL | https://pubchem.ncbi.nlm.nih.gov | |
Description | Data deposited in or computed by PubChem | |
CAS No. |
4510-83-2 | |
Record name | Bis(dibenziodonium) sulphate | |
Source | ChemIDplus | |
URL | https://pubchem.ncbi.nlm.nih.gov/substance/?source=chemidplus&sourceid=0004510832 | |
Description | ChemIDplus is a free, web search system that provides access to the structure and nomenclature authority files used for the identification of chemical substances cited in National Library of Medicine (NLM) databases, including the TOXNET system. | |
Record name | Dibenziodolium, sulfate (2:1) | |
Source | EPA DSSTox | |
URL | https://comptox.epa.gov/dashboard/DTXSID701044278 | |
Description | DSSTox provides a high quality public chemistry resource for supporting improved predictive toxicology. | |
Record name | Bis(dibenziodonium) sulphate | |
Source | European Chemicals Agency (ECHA) | |
URL | https://echa.europa.eu/substance-information/-/substanceinfo/100.022.574 | |
Description | The European Chemicals Agency (ECHA) is an agency of the European Union which is the driving force among regulatory authorities in implementing the EU's groundbreaking chemicals legislation for the benefit of human health and the environment as well as for innovation and competitiveness. | |
Explanation | Use of the information, documents and data from the ECHA website is subject to the terms and conditions of this Legal Notice, and subject to other binding limitations provided for under applicable law, the information, documents and data made available on the ECHA website may be reproduced, distributed and/or used, totally or in part, for non-commercial purposes provided that ECHA is acknowledged as the source: "Source: European Chemicals Agency, http://echa.europa.eu/". Such acknowledgement must be included in each copy of the material. ECHA permits and encourages organisations and individuals to create links to the ECHA website under the following cumulative conditions: Links can only be made to webpages that provide a link to the Legal Notice page. | |
Q1: What is the primary mechanism of action of Diphenyleneiodonium sulfate (DPI)?
A1: DPI is a well-known inhibitor of flavoproteins, specifically targeting NADPH oxidase (NOX) enzymes. [, , , , , ] It acts by inhibiting the electron transfer within these enzymes, preventing the formation of reactive oxygen species (ROS) like superoxide. [, ]
Q2: What are the downstream effects of DPI's inhibition of NADPH oxidase?
A2: Inhibition of NOX by DPI has been shown to prevent various cellular processes driven by ROS. For instance, DPI effectively blocks Angiotensin II-induced MCP-1 expression in mesangial cells by inhibiting NOX-derived ROS. [] Similarly, DPI prevents early alcohol-induced liver injury by inhibiting free radical formation via NOX, consequently suppressing NF-kappaB activation and TNF-alpha expression. []
Q3: How does DPI impact the biotransformation of Glyceryl trinitrate (GTN)?
A3: DPI significantly inhibits the biotransformation of GTN, a nitric oxide (NO) donor used in the treatment of angina. [, ] This inhibition of GTN bioactivation by DPI is observed through decreased cyclic GMP accumulation and reduced vasodilation in rat aorta. [, ] Interestingly, DPI also affects the biotransformation of other organic nitrates like D-isoidide dinitrate, but not its L-enantiomer. []
Q4: What is the role of DPI in understanding organic nitrate tolerance?
A4: While increased NOX activity was proposed to contribute to organic nitrate tolerance, studies using DPI challenge this hypothesis. DPI showed similar inhibitory effects on GTN-induced relaxation in both control and tolerant rat aorta. [] This finding, coupled with unchanged NADPH-cytochrome P450 reductase expression in tolerant tissues, suggests factors beyond NOX-mediated superoxide generation are involved in tolerance development. []
Q5: Are there alternative mechanisms through which DPI exerts its effects?
A5: While primarily known for NOX inhibition, DPI can influence other pathways. For example, DPI can modestly increase the sensitivity of rat aorta to the NO donor DEA NONOate, suggesting potential interaction with NO signaling pathways. []
Q6: How does DPI impact the inflammatory response in the liver?
A6: DPI has been shown to prevent the ethanol-induced sensitization of Kupffer cells to endotoxin, a key event in alcoholic liver injury. [] This protective effect is linked to DPI's ability to inhibit the NADPH oxidase-mediated increase in IRAK expression, a signaling molecule involved in TLR4 activation. [] Furthermore, DPI can reduce the upregulation of several Toll-like receptors (TLRs) in the liver of ethanol-fed mice, demonstrating a broader impact on inflammatory pathways. []
Q7: Does DPI affect neutrophil extracellular trap (NET) formation?
A7: Interestingly, DPI effectively inhibits phorbol 12-myristate 13-acetate (PMA)-stimulated NET formation in neutrophils, regardless of their G6PD status. [] This finding suggests the involvement of NADPH oxidase in NETosis and highlights DPI's ability to modulate neutrophil function. []
Disclaimer and Information on In-Vitro Research Products
Please be aware that all articles and product information presented on BenchChem are intended solely for informational purposes. The products available for purchase on BenchChem are specifically designed for in-vitro studies, which are conducted outside of living organisms. In-vitro studies, derived from the Latin term "in glass," involve experiments performed in controlled laboratory settings using cells or tissues. It is important to note that these products are not categorized as medicines or drugs, and they have not received approval from the FDA for the prevention, treatment, or cure of any medical condition, ailment, or disease. We must emphasize that any form of bodily introduction of these products into humans or animals is strictly prohibited by law. It is essential to adhere to these guidelines to ensure compliance with legal and ethical standards in research and experimentation.