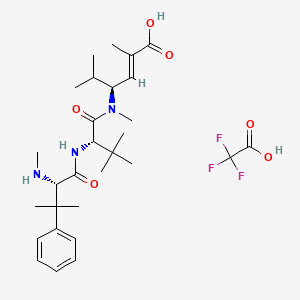
Taltobulin trifluoroacetate
Overview
Description
Preparation Methods
The synthesis of Taltobulin trifluoroacetate involves several steps. One method begins with the Friedel-Crafts condensation of dimethylacrylic acid with benzene in the presence of aluminum chloride to produce 3-methyl-3-phenylbutanoic acid. This acid is then activated as a mixed anhydride by treatment with pivaloyl chloride and triethylamine, followed by coupling with the lithium salt of (S)-4-isopropyl-2-oxazolidinone to furnish the chiral N-acyl oxazolidinone. Subsequent steps include diastereoselective introduction of an azido group, catalytic hydrogenation, and several other reactions to yield the final tripeptide .
Chemical Reactions Analysis
Taltobulin trifluoroacetate undergoes various chemical reactions, primarily involving its antimicrotubule activity. It inhibits the polymerization of tubulin, leading to the disruption of microtubule organization within cells. This disruption induces mitotic arrest and apoptosis . Common reagents used in these reactions include tubulin and various cell lines for in vitro studies .
Scientific Research Applications
Taltobulin trifluoroacetate has significant applications in scientific research, particularly in the fields of chemistry, biology, and medicine. It is used as a cytotoxic agent in antibody-drug conjugates for targeted cancer therapy . Its ability to circumvent P-glycoprotein-mediated resistance makes it valuable in studying drug resistance mechanisms in cancer cells . Additionally, it has been investigated for its potential to inhibit the growth of various tumor cell lines, including leukemia, ovarian, non-small cell lung cancer, breast, colon, and melanoma .
Mechanism of Action
The mechanism of action of Taltobulin trifluoroacetate involves its binding to the vinca domain between the alpha- and beta-subunits of tubulin. This binding inhibits tubulin polymerization, leading to the disruption of microtubule dynamics. The compound induces mitotic arrest and apoptosis by interfering with the normal function of microtubules during cell division . It also activates both the extracellular signal-regulated kinases and phosphoinositide 3-kinase/AKT signaling pathways, promoting cell viability and reducing apoptosis in certain contexts .
Comparison with Similar Compounds
Taltobulin trifluoroacetate is similar to other antimicrotubule agents such as docetaxel and paclitaxel. it is unique in its ability to circumvent P-glycoprotein-mediated resistance, which is a common issue with other antimicrotubule agents . Similar compounds include hemiasterlin, docetaxel, and paclitaxel . Each of these compounds has distinct molecular signatures and mechanisms of action, but this compound stands out due to its effectiveness against drug-resistant cancer cells .
Properties
IUPAC Name |
(E,4S)-4-[[(2S)-3,3-dimethyl-2-[[(2S)-3-methyl-2-(methylamino)-3-phenylbutanoyl]amino]butanoyl]-methylamino]-2,5-dimethylhex-2-enoic acid;2,2,2-trifluoroacetic acid | |
---|---|---|
Source | PubChem | |
URL | https://pubchem.ncbi.nlm.nih.gov | |
Description | Data deposited in or computed by PubChem | |
InChI |
InChI=1S/C27H43N3O4.C2HF3O2/c1-17(2)20(16-18(3)25(33)34)30(10)24(32)22(26(4,5)6)29-23(31)21(28-9)27(7,8)19-14-12-11-13-15-19;3-2(4,5)1(6)7/h11-17,20-22,28H,1-10H3,(H,29,31)(H,33,34);(H,6,7)/b18-16+;/t20-,21-,22-;/m1./s1 | |
Source | PubChem | |
URL | https://pubchem.ncbi.nlm.nih.gov | |
Description | Data deposited in or computed by PubChem | |
InChI Key |
SHWPCKJNFBDPFA-LPWSJWOVSA-N | |
Source | PubChem | |
URL | https://pubchem.ncbi.nlm.nih.gov | |
Description | Data deposited in or computed by PubChem | |
Canonical SMILES |
CC(C)C(C=C(C)C(=O)O)N(C)C(=O)C(C(C)(C)C)NC(=O)C(C(C)(C)C1=CC=CC=C1)NC.C(=O)(C(F)(F)F)O | |
Source | PubChem | |
URL | https://pubchem.ncbi.nlm.nih.gov | |
Description | Data deposited in or computed by PubChem | |
Isomeric SMILES |
CC(C)[C@@H](/C=C(\C)/C(=O)O)N(C)C(=O)[C@H](C(C)(C)C)NC(=O)[C@H](C(C)(C)C1=CC=CC=C1)NC.C(=O)(C(F)(F)F)O | |
Source | PubChem | |
URL | https://pubchem.ncbi.nlm.nih.gov | |
Description | Data deposited in or computed by PubChem | |
Molecular Formula |
C29H44F3N3O6 | |
Source | PubChem | |
URL | https://pubchem.ncbi.nlm.nih.gov | |
Description | Data deposited in or computed by PubChem | |
Molecular Weight |
587.7 g/mol | |
Source | PubChem | |
URL | https://pubchem.ncbi.nlm.nih.gov | |
Description | Data deposited in or computed by PubChem | |
Retrosynthesis Analysis
AI-Powered Synthesis Planning: Our tool employs the Template_relevance Pistachio, Template_relevance Bkms_metabolic, Template_relevance Pistachio_ringbreaker, Template_relevance Reaxys, Template_relevance Reaxys_biocatalysis model, leveraging a vast database of chemical reactions to predict feasible synthetic routes.
One-Step Synthesis Focus: Specifically designed for one-step synthesis, it provides concise and direct routes for your target compounds, streamlining the synthesis process.
Accurate Predictions: Utilizing the extensive PISTACHIO, BKMS_METABOLIC, PISTACHIO_RINGBREAKER, REAXYS, REAXYS_BIOCATALYSIS database, our tool offers high-accuracy predictions, reflecting the latest in chemical research and data.
Strategy Settings
Precursor scoring | Relevance Heuristic |
---|---|
Min. plausibility | 0.01 |
Model | Template_relevance |
Template Set | Pistachio/Bkms_metabolic/Pistachio_ringbreaker/Reaxys/Reaxys_biocatalysis |
Top-N result to add to graph | 6 |
Feasible Synthetic Routes
Disclaimer and Information on In-Vitro Research Products
Please be aware that all articles and product information presented on BenchChem are intended solely for informational purposes. The products available for purchase on BenchChem are specifically designed for in-vitro studies, which are conducted outside of living organisms. In-vitro studies, derived from the Latin term "in glass," involve experiments performed in controlled laboratory settings using cells or tissues. It is important to note that these products are not categorized as medicines or drugs, and they have not received approval from the FDA for the prevention, treatment, or cure of any medical condition, ailment, or disease. We must emphasize that any form of bodily introduction of these products into humans or animals is strictly prohibited by law. It is essential to adhere to these guidelines to ensure compliance with legal and ethical standards in research and experimentation.