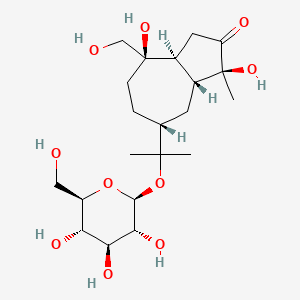
Atractyloside A
Overview
Description
Atractyloside A is a natural, toxic glycoside found in various plant species, particularly in the daisy family. It is known for its potent inhibitory effects on the mitochondrial ADP/ATP translocase, which disrupts cellular energy metabolism . This compound has been historically used for therapeutic, religious, and toxic purposes .
Mechanism of Action
Target of Action
Atractyloside A (AA) primarily targets the Adenine Nucleotide Translocase (ANT) . ANT is responsible for transporting ADP from the cytoplasm to the mitochondrial matrix, providing raw materials for ATP synthesis via oxidative phosphorylation .
Mode of Action
AA acts as a competitive inhibitor binding to ANT . This binding makes ANT vulnerable to transport ADP, thereby reducing ATP synthesis . The blockage of ANT by AA may increase the ADP/ATP ratio .
Biochemical Pathways
The increased ADP/ATP ratio activates the AMPK-mTORC1-autophagy signaling pathway , promoting lipid degradation in steatosis hepatocytes . Additionally, AA has been found to inhibit the p38 MAPK pathway , which is involved in cellular responses to stress and inflammation.
Pharmacokinetics
Atractylenolides, including AA, are rapidly absorbed but slowly metabolized . This slow metabolism could potentially lead to a longer duration of action. Due to the inhibitory effects of atractylenolides on metabolic enzymes, caution is advised when combining atractylenolides with other drugs .
Result of Action
AA has been found to have multiple effects at the molecular and cellular levels. It can increase body weight, intestinal propulsion, and levels of motilin (MTL), gastrin (GAS), and c-Kit, while decreasing gastric residual volume and intestinal tissue damage . Furthermore, AA has been shown to protect multiple organs by modulating oxidative stress, attenuating the inflammatory response, activating anti-apoptotic signaling pathways, and inhibiting cell apoptosis .
Action Environment
The concentration of AA found in plants is dependent upon the species, season, and origin . For example, the AA content measured in dried Atractylis gummifera between Sardinia, Italy and Sicily, Italy revealed a higher content in the Sicilian region by nearly a factor of five, and a higher content in colder months across both regions . Therefore, environmental factors can influence the compound’s action, efficacy, and stability.
Biochemical Analysis
Biochemical Properties
Atractyloside A acts as an effective ADP/ATP translocase inhibitor which eventually halts ADP and ATP exchange and the cell dies due to lack of energy . The chemical structure and charge distribution of this compound is similar to that of ADP .
Cellular Effects
This compound has shown potential antiviral properties against the influenza B virus . It not only possesses anti-influenza B virus infection effects in vivo and in vitro but also can regulate macrophage polarization to the M2 type, which can effectively attenuate the damage caused by influenza B virus infection . Atractylenolides, including this compound, can protect multiple organs by modulating oxidative stress, attenuating the inflammatory response, activating anti-apoptotic signaling pathways, and inhibiting cell apoptosis .
Molecular Mechanism
The anti-cancer activity of atractylenolides can be attributed to their influence on the JAK2/STAT3 signaling pathway . Additionally, the TLR4/NF-κB, PI3K/Akt, and MAPK signaling pathways primarily mediate the anti-inflammatory effects of these compounds .
Temporal Effects in Laboratory Settings
This compound has been shown to inhibit the proliferation of non-small cell lung cancer cells . Due to the inhibitory effects of atractylenolides on metabolic enzymes, it is necessary to pay attention to the possible side effects of combining atractylenolides with other drugs .
Metabolic Pathways
In a cytochrome P450 (CYP450)-mimetic oxidation model, atractylenolide-II is oxidized to atractylenolide-III, and atractylenolide-III is converted to atractylenolide-I via dehydration . This observation indicates that there is a mutual conversion relationship between the atractylenolides .
Transport and Distribution
This compound inhibits the transport of ADP into and ATP out of the mitochondria by competing with ADP for binding to the carrier .
Preparation Methods
Synthetic Routes and Reaction Conditions
The synthesis of Atractyloside A involves complex organic reactions. One common method includes the extraction from the rhizomes of Atractylis gummifera, followed by purification using high-performance liquid chromatography . The reaction conditions typically involve maintaining a controlled temperature and pH to ensure the stability of the compound during extraction and purification.
Industrial Production Methods
Industrial production of this compound is primarily based on the extraction from natural sources. The process involves harvesting the plant material, drying, and then extracting the compound using solvents. The extract is then purified using chromatographic techniques to obtain high-purity this compound .
Chemical Reactions Analysis
Types of Reactions
Atractyloside A undergoes several types of chemical reactions, including:
Oxidation: This reaction can convert this compound into other related compounds.
Reduction: This reaction can modify the glycosidic bonds within the molecule.
Substitution: This reaction can replace functional groups within the molecule with other groups.
Common Reagents and Conditions
Common reagents used in these reactions include oxidizing agents like potassium permanganate, reducing agents like sodium borohydride, and various acids and bases for substitution reactions. The conditions often involve controlled temperatures and pH levels to ensure the desired reaction pathway .
Major Products Formed
The major products formed from these reactions include various oxidized, reduced, and substituted derivatives of this compound. These derivatives often retain the core glycoside structure but exhibit different biological activities .
Scientific Research Applications
Atractyloside A has a wide range of scientific research applications:
Chemistry: Used as a model compound to study glycoside reactions and interactions.
Biology: Investigated for its effects on mitochondrial function and cellular energy metabolism.
Medicine: Explored for its potential therapeutic effects and toxicological properties.
Industry: Used in the development of bioactive compounds and as a reference standard in analytical chemistry
Comparison with Similar Compounds
Similar Compounds
Carboxyatractyloside: Another toxic glycoside with similar inhibitory effects on the ADP/ATP translocase.
Atractylenolide I, II, and III: Compounds derived from Atractylodes macrocephala with various pharmacological activities.
Uniqueness
Atractyloside A is unique due to its potent inhibitory effects on mitochondrial function and its historical significance in traditional medicine. Unlike other similar compounds, it has a well-documented toxicological profile and specific applications in scientific research .
Properties
IUPAC Name |
(3S,3aR,5R,8R,8aS)-3,8-dihydroxy-8-(hydroxymethyl)-3-methyl-5-[2-[(2S,3R,4S,5S,6R)-3,4,5-trihydroxy-6-(hydroxymethyl)oxan-2-yl]oxypropan-2-yl]-3a,4,5,6,7,8a-hexahydro-1H-azulen-2-one | |
---|---|---|
Source | PubChem | |
URL | https://pubchem.ncbi.nlm.nih.gov | |
Description | Data deposited in or computed by PubChem | |
InChI |
InChI=1S/C21H36O10/c1-19(2,31-18-17(27)16(26)15(25)13(8-22)30-18)10-4-5-21(29,9-23)12-7-14(24)20(3,28)11(12)6-10/h10-13,15-18,22-23,25-29H,4-9H2,1-3H3/t10-,11-,12+,13-,15-,16+,17-,18+,20+,21+/m1/s1 | |
Source | PubChem | |
URL | https://pubchem.ncbi.nlm.nih.gov | |
Description | Data deposited in or computed by PubChem | |
InChI Key |
QNBLVYVBWDIWDM-BSLJOXIBSA-N | |
Source | PubChem | |
URL | https://pubchem.ncbi.nlm.nih.gov | |
Description | Data deposited in or computed by PubChem | |
Canonical SMILES |
CC1(C2CC(CCC(C2CC1=O)(CO)O)C(C)(C)OC3C(C(C(C(O3)CO)O)O)O)O | |
Source | PubChem | |
URL | https://pubchem.ncbi.nlm.nih.gov | |
Description | Data deposited in or computed by PubChem | |
Isomeric SMILES |
C[C@@]1([C@@H]2C[C@@H](CC[C@@]([C@H]2CC1=O)(CO)O)C(C)(C)O[C@H]3[C@@H]([C@H]([C@@H]([C@H](O3)CO)O)O)O)O | |
Source | PubChem | |
URL | https://pubchem.ncbi.nlm.nih.gov | |
Description | Data deposited in or computed by PubChem | |
Molecular Formula |
C21H36O10 | |
Source | PubChem | |
URL | https://pubchem.ncbi.nlm.nih.gov | |
Description | Data deposited in or computed by PubChem | |
DSSTOX Substance ID |
DTXSID901347606 | |
Record name | Atractyloside A | |
Source | EPA DSSTox | |
URL | https://comptox.epa.gov/dashboard/DTXSID901347606 | |
Description | DSSTox provides a high quality public chemistry resource for supporting improved predictive toxicology. | |
Molecular Weight |
448.5 g/mol | |
Source | PubChem | |
URL | https://pubchem.ncbi.nlm.nih.gov | |
Description | Data deposited in or computed by PubChem | |
CAS No. |
126054-77-1 | |
Record name | Atractyloside A | |
Source | EPA DSSTox | |
URL | https://comptox.epa.gov/dashboard/DTXSID901347606 | |
Description | DSSTox provides a high quality public chemistry resource for supporting improved predictive toxicology. | |
Q1: What is the primary biological target of Atractyloside A (AA)?
A1: AA is a potent inhibitor of the Adenine Nucleotide Translocator (ANT), also known as the ADP/ATP carrier, located in the inner mitochondrial membrane [, , ].
Q2: How does AA exert its inhibitory effect on the ANT?
A2: AA binds with high affinity to the ANT, blocking the exchange of ADP and ATP across the mitochondrial membrane. This disrupts the mitochondrial membrane potential and inhibits oxidative phosphorylation [, , ].
Q3: What are the downstream consequences of AA binding to ANT?
A3: By inhibiting ANT, AA leads to a depletion of intracellular ATP, disruption of cellular energy balance, and ultimately, cell death. This toxicity is particularly pronounced in organs with high energy demands, such as the liver and kidneys [, , , ].
Q4: Are there other potential targets for AA besides ANT?
A4: Recent research suggests that AA might interact with other targets within the cell, including Nucleoside Diphosphate Kinase (NDPK), potentially contributing to its overall toxicity []. Further research is needed to fully elucidate these interactions.
Q5: How does the mechanism of action of AA differ from that of bongkrekic acid, another ANT inhibitor?
A5: While both AA and bongkrekic acid inhibit ANT, they do so by binding to distinct sites on the carrier protein. AA binds to the outer (cytosolic) face of the ANT, while bongkrekic acid binds to the inner (matrix) face. This difference in binding site location is reflected in their distinct inhibitory profiles [, ].
Q6: What is the molecular formula and weight of this compound?
A6: The molecular formula of AA is C30H46O13S2, and its molecular weight is 686.8 g/mol.
Q7: Is there any spectroscopic data available to help characterize this compound?
A7: Yes, various spectroscopic techniques have been employed to characterize AA. These include UV-Vis spectrophotometry, Nuclear Magnetic Resonance (NMR) spectroscopy, and mass spectrometry (MS) [, , , , ].
Q8: Does this compound exhibit any catalytic properties?
A8: AA is not known to possess catalytic properties. Its primary mode of action is through binding and inhibiting the ANT.
Q9: Have any computational studies been conducted on this compound?
A10: While detailed computational studies specifically on AA are limited in the provided research, computational methods like molecular docking have been used to study the interaction of similar compounds with ANT, providing insights into binding modes and potential for drug development [, ].
Q10: How does the structure of this compound contribute to its activity?
A11: Specific structural features of AA, particularly its diterpenoid core and the presence of sulfate groups, are crucial for its high-affinity binding to the ANT. Modifications to these key structural elements can significantly impact its inhibitory potency and selectivity [, ].
Q11: Are there specific formulation strategies to enhance the stability or bioavailability of this compound?
A11: While specific formulation strategies for AA are not extensively discussed in the provided literature, research on related compounds suggests that encapsulation techniques, such as liposomes or nanoparticles, could potentially be explored to improve its stability, solubility, or bioavailability.
Q12: Are there specific SHE regulations regarding the handling and disposal of this compound?
A12: Given the known toxicity of AA, appropriate safety measures and adherence to established laboratory guidelines for handling hazardous substances are crucial. Researchers should consult relevant safety data sheets and regulations for proper handling, storage, and disposal procedures.
Q13: What are the common in vitro and in vivo models used to study this compound's effects?
A15: Common in vitro models include isolated mitochondria [, , ] and cell lines [, , ]. In vivo, rodent models are often employed to investigate AA-induced toxicity, particularly focusing on liver and kidney damage [, , ].
Q14: What are the primary toxicological concerns associated with this compound exposure?
A17: AA is a known hepatotoxin and nephrotoxin. Its consumption can lead to severe liver and kidney damage, potentially resulting in organ failure and death. Even low doses can be harmful, and there is no known antidote for AA poisoning [, , , ].
Q15: What analytical methods are commonly used to detect and quantify this compound?
A20: High-Performance Liquid Chromatography (HPLC) coupled with various detection methods, including Diode Array Detection (DAD) and Mass Spectrometry (MS), is widely employed for the analysis of AA in biological samples [, , , ].
Q16: What is the environmental impact of this compound and its degradation products?
A16: Specific information regarding the environmental fate and ecotoxicological effects of AA and its degradation products is limited in the provided research and would require further investigation.
Q17: Are the analytical methods used for this compound determination validated?
A22: Yes, several studies have validated analytical methods, such as HPLC-MS/MS, for quantifying AA in various matrices, including plant material, blood, and plasma. These validation procedures typically involve assessing parameters like linearity, accuracy, precision, and recovery [, , ].
Disclaimer and Information on In-Vitro Research Products
Please be aware that all articles and product information presented on BenchChem are intended solely for informational purposes. The products available for purchase on BenchChem are specifically designed for in-vitro studies, which are conducted outside of living organisms. In-vitro studies, derived from the Latin term "in glass," involve experiments performed in controlled laboratory settings using cells or tissues. It is important to note that these products are not categorized as medicines or drugs, and they have not received approval from the FDA for the prevention, treatment, or cure of any medical condition, ailment, or disease. We must emphasize that any form of bodily introduction of these products into humans or animals is strictly prohibited by law. It is essential to adhere to these guidelines to ensure compliance with legal and ethical standards in research and experimentation.