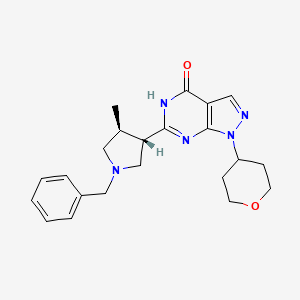
PDE-9 inhibitor
Overview
Description
Phosphodiesterase-9 (PDE-9) inhibitors are a class of drugs that work by inhibiting the activity of PDE-9 . The cardioprotective effects of natriuretic peptides (NPs), which are released in response to increased ventricular stretch in the syndrome of heart failure (HF), are mediated by the secondary messenger cyclic guanosine monophosphate (cGMP) . PDE-9 inhibitors have been studied for their potential to treat diabetes, neurodegenerative disorders, cardiovascular diseases, and erectile dysfunction .
Synthesis Analysis
A computational study on selective PDE-9 inhibitors has been conducted to elucidate the differences in their interaction patterns in the presence of different metal systems such as Zn/Mg, Mg/Mg, and Zn/Zn . The study selected highly potent previously reported selective PDE-9 inhibitors BAY73-6691R, BAY73-6691S, 28r, 28s, 3r, 3s, PF-0447943, PF-4181366, and 4r .
Molecular Structure Analysis
The crystal structure of the PDE9A catalytic domain complexed with an orally bioavailable small molecule inhibitor (PDB id – 9Q9) was obtained from PBD . Seven different structure-based pharmacophore models were developed from 9Q9, a PDE9 inhibitor complexed with the 3D structure of the protein (PDB 6A3N) .
Chemical Reactions Analysis
PDE inhibitors are characterized by the compliance-limiting adverse reactions . Efforts are directed to appropriately adjusting the dose regimens and conducting structure-activity relationship studies to determine the effect of structural features on the safety profile .
Physical And Chemical Properties Analysis
PDE inhibitors are a class of agents acting on specific phosphodiesterase enzymes in target cells . They prevent the degradation of cAMP or cGMP, exhibiting smooth muscle relaxation, vasodilatory, and bronchodilatory effects .
Scientific Research Applications
Neurodegeneration and Alzheimer’s Disease
PDE-9 inhibitors have shown promise in the field of neurodegeneration, particularly in the treatment of Alzheimer’s disease . PDE-9 inhibition promotes neurotransmitter release, amelioration of microvascular dysfunction, and neuronal plasticity . This could potentially help contrast neurodegeneration and improve symptoms of Alzheimer’s disease .
Synaptic Plasticity
PDE-9 enzyme hydrolyzes cGMP, which is involved in the regulation of synaptic plasticity through the NMDA pathway . The inhibition of PDE-9 leads to elevated cGMP levels, causing enhanced NMDA signaling and thus contributing to an increase in synaptic plasticity and stabilization .
Obesity
PDE-9 inhibitors are under investigation for the treatment of obesity . While the exact mechanisms are still being studied, it is believed that the modulation of cGMP levels could potentially influence metabolic processes and help manage obesity .
Hepatic Fibrosis
Hepatic fibrosis, a condition characterized by the excessive accumulation of extracellular matrix proteins including collagen, is another potential application for PDE-9 inhibitors . The role of cGMP in liver function and fibrosis is a growing area of research .
Heart Failure
Heart failure is a complex clinical syndrome that results from any structural or functional impairment of ventricular filling or ejection of blood. PDE-9 inhibitors are being investigated for their potential role in the treatment of heart failure .
Sickle Cell Anemia
In mouse models of sickle cell disease (SCD), inhibition of PDE9 had beneficial effects in the prophylactic reduction of crisis . Co-administration of PDE9 inhibitor with hydroxyurea led to a significant reduction in vaso-occlusion associated with sickle cell disease .
Mechanism of Action
Target of Action
Phosphodiesterase 9 (PDE9) inhibitors are a class of drugs that work by inhibiting the activity of PDE9 . The primary targets of PDE9 inhibitors are the PDE9 enzymes, which are key regulators of the cyclic nucleotide signal transduction system . These enzymes are involved in the breakdown of phosphodiester bonds in various cyclic nucleotides, thereby regulating signal transduction .
Mode of Action
PDE9 inhibitors interact with their targets, the PDE9 enzymes, by inhibiting their activity . This inhibition leads to an increase in the levels of cyclic guanosine monophosphate (cGMP), a second messenger involved in various biological responses . The increased cGMP levels enhance signaling pathways, contributing to various biological effects .
Biochemical Pathways
The inhibition of PDE9 leads to elevated cGMP levels, causing enhanced signaling through the NMDA pathway . This pathway is involved in the regulation of synaptic plasticity via activation of calcium/calmodulin-dependent neuronal NO synthases in the postsynaptic neurons . The increased cGMP levels and enhanced NMDA signaling contribute to an increase in synaptic plasticity and stabilization .
Pharmacokinetics
While specific ADME (Absorption, Distribution, Metabolism, Excretion) properties of PDE9 inhibitors may vary between different compounds, these drugs generally exhibit good bioavailability . The pharmacokinetic properties of these drugs are still under investigation, and efforts are being made to adjust dose regimens and conduct structure-activity relationship studies to determine the effect of structural features on the safety profile .
Result of Action
The molecular and cellular effects of PDE9 inhibitors’ action include increased fetal hemoglobin (HbF) expression and reduced hemolysis and sickling of red blood cells (RBCs), which can lead to a reduction in painful vaso-occlusive crises . In addition, these inhibitors have been shown to reduce obesity and cardiometabolic syndrome in mice .
Action Environment
The action, efficacy, and stability of PDE9 inhibitors can be influenced by various environmental factors. For instance, the presence of other drugs (such as hydroxyurea) can affect the action of PDE9 inhibitors . Furthermore, the physiological environment, such as the state of the disease in the patient, can also influence the efficacy of these drugs .
Safety and Hazards
Future Directions
PDE-9 inhibitors are under investigation for the treatment of obesity, hepatic fibrosis, Alzheimer’s disease, schizophrenia, other psychotic disorders, heart failure, and sickle cell anemia . The ongoing development predominantly concentrates on central nervous system diseases, such as schizophrenia, Alzheimer’s disease, Parkinson’s disease and fragile X syndrome . Notable advancements are also being made in mycobacterial infections, HIV and Duchenne muscular dystrophy .
properties
IUPAC Name |
6-[(3S,4S)-1-benzyl-4-methylpyrrolidin-3-yl]-1-(oxan-4-yl)-5H-pyrazolo[3,4-d]pyrimidin-4-one | |
---|---|---|
Source | PubChem | |
URL | https://pubchem.ncbi.nlm.nih.gov | |
Description | Data deposited in or computed by PubChem | |
InChI |
InChI=1S/C22H27N5O2/c1-15-12-26(13-16-5-3-2-4-6-16)14-19(15)20-24-21-18(22(28)25-20)11-23-27(21)17-7-9-29-10-8-17/h2-6,11,15,17,19H,7-10,12-14H2,1H3,(H,24,25,28)/t15-,19-/m1/s1 | |
Source | PubChem | |
URL | https://pubchem.ncbi.nlm.nih.gov | |
Description | Data deposited in or computed by PubChem | |
InChI Key |
RVEJWGYZBXCGGM-DNVCBOLYSA-N | |
Source | PubChem | |
URL | https://pubchem.ncbi.nlm.nih.gov | |
Description | Data deposited in or computed by PubChem | |
Canonical SMILES |
CC1CN(CC1C2=NC3=C(C=NN3C4CCOCC4)C(=O)N2)CC5=CC=CC=C5 | |
Source | PubChem | |
URL | https://pubchem.ncbi.nlm.nih.gov | |
Description | Data deposited in or computed by PubChem | |
Isomeric SMILES |
C[C@@H]1CN(C[C@H]1C2=NC3=C(C=NN3C4CCOCC4)C(=O)N2)CC5=CC=CC=C5 | |
Source | PubChem | |
URL | https://pubchem.ncbi.nlm.nih.gov | |
Description | Data deposited in or computed by PubChem | |
Molecular Formula |
C22H27N5O2 | |
Source | PubChem | |
URL | https://pubchem.ncbi.nlm.nih.gov | |
Description | Data deposited in or computed by PubChem | |
DSSTOX Substance ID |
DTXSID10648755 | |
Record name | 6-[(3S,4S)-1-Benzyl-4-methylpyrrolidin-3-yl]-1-(oxan-4-yl)-1,2-dihydro-4H-pyrazolo[3,4-d]pyrimidin-4-one | |
Source | EPA DSSTox | |
URL | https://comptox.epa.gov/dashboard/DTXSID10648755 | |
Description | DSSTox provides a high quality public chemistry resource for supporting improved predictive toxicology. | |
Molecular Weight |
393.5 g/mol | |
Source | PubChem | |
URL | https://pubchem.ncbi.nlm.nih.gov | |
Description | Data deposited in or computed by PubChem | |
Product Name |
PDE-9 inhibitor | |
CAS RN |
1082743-70-1 | |
Record name | 1,5-Dihydro-6-[(3S,4S)-4-methyl-1-(phenylmethyl)-3-pyrrolidinyl]-1-(tetrahydro-2H-pyran-4-yl)-4H-pyrazolo[3,4-d]pyrimidin-4-one | |
Source | CAS Common Chemistry | |
URL | https://commonchemistry.cas.org/detail?cas_rn=1082743-70-1 | |
Description | CAS Common Chemistry is an open community resource for accessing chemical information. Nearly 500,000 chemical substances from CAS REGISTRY cover areas of community interest, including common and frequently regulated chemicals, and those relevant to high school and undergraduate chemistry classes. This chemical information, curated by our expert scientists, is provided in alignment with our mission as a division of the American Chemical Society. | |
Explanation | The data from CAS Common Chemistry is provided under a CC-BY-NC 4.0 license, unless otherwise stated. | |
Record name | 6-[(3S,4S)-1-Benzyl-4-methylpyrrolidin-3-yl]-1-(oxan-4-yl)-1,2-dihydro-4H-pyrazolo[3,4-d]pyrimidin-4-one | |
Source | EPA DSSTox | |
URL | https://comptox.epa.gov/dashboard/DTXSID10648755 | |
Description | DSSTox provides a high quality public chemistry resource for supporting improved predictive toxicology. | |
Q & A
Q1: What is the primary mechanism of action for PDE-9 inhibitors and how does this relate to their potential therapeutic benefit in sickle cell disease (SCD)?
A1: PDE-9 inhibitors specifically target and block the activity of phosphodiesterase-9A (PDE-9A), an enzyme responsible for degrading cyclic guanosine monophosphate (cGMP) []. By inhibiting PDE-9A, these compounds increase intracellular cGMP levels. This is particularly relevant in SCD as cGMP plays a role in regulating vascular tone and inflammation. Increased cGMP levels can lead to vasodilation and reduced leukocyte adhesion, potentially mitigating vaso-occlusive crises, a hallmark of SCD [].
Q2: The research mentions the use of PF-04447943, a specific PDE-9 inhibitor. What is known about its in vivo effects in preclinical models of SCD?
A2: In the Townes mouse model of SCD, prophylactic treatment with PF-04447943 in combination with hydroxyurea demonstrated a significant reduction in neutrophil adhesion and neutrophil-platelet aggregates in the cremaster muscle microvasculature []. This suggests that PF-04447943, particularly when combined with hydroxyurea, might be beneficial in preventing vaso-occlusive episodes in SCD.
Q3: Were there any synergistic effects observed when combining PF-04447943 with hydroxyurea in preclinical models?
A3: Yes, the study using the Townes mouse model of SCD showed that co-administration of PF-04447943 and hydroxyurea led to a more pronounced reduction in neutrophil adhesion and neutrophil-platelet aggregates compared to either treatment alone []. This suggests a potential synergistic effect between these two compounds. The mechanism behind this synergy might be linked to their combined effects on nitric oxide bioavailability and cGMP signaling.
Q4: Aside from SCD, are there other potential therapeutic applications being investigated for PDE-9 inhibitors?
A4: Yes, research suggests that PDE-9 inhibitors may have therapeutic potential for other conditions. One study explored the effects of PF-04447943 on olfactory memory in mice using the Social Transmission of Food Preference test []. Other research investigated the impact of BAY60-7550 (a selective PDE-2 inhibitor), Sildenafil (a PDE-5 inhibitor), and PF-04447943 on learning and memory in a passive avoidance test using mice []. These studies indicate broader research interest in PDE-9 inhibitors for cognitive function and other potential therapeutic areas.
Disclaimer and Information on In-Vitro Research Products
Please be aware that all articles and product information presented on BenchChem are intended solely for informational purposes. The products available for purchase on BenchChem are specifically designed for in-vitro studies, which are conducted outside of living organisms. In-vitro studies, derived from the Latin term "in glass," involve experiments performed in controlled laboratory settings using cells or tissues. It is important to note that these products are not categorized as medicines or drugs, and they have not received approval from the FDA for the prevention, treatment, or cure of any medical condition, ailment, or disease. We must emphasize that any form of bodily introduction of these products into humans or animals is strictly prohibited by law. It is essential to adhere to these guidelines to ensure compliance with legal and ethical standards in research and experimentation.