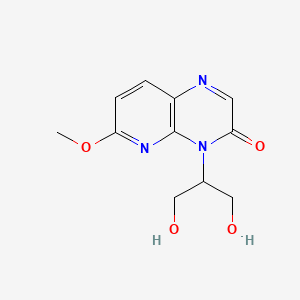
beta-lactamase-IN-1
Overview
Description
Beta-lactamase-IN-1 is a compound known for its ability to inhibit beta-lactamase enzymes. Beta-lactamase enzymes are produced by bacteria and are responsible for conferring resistance to beta-lactam antibiotics, such as penicillins and cephalosporins. By inhibiting these enzymes, this compound helps restore the efficacy of beta-lactam antibiotics against resistant bacterial strains .
Mechanism of Action
Target of Action
Beta-lactamase-IN-1, also known as 4-(1,3-Dihydroxypropan-2-yl)-6-methoxypyrido[2,3-b]pyrazin-3(4H)-one, primarily targets β-lactamases . β-lactamases are a diverse class of enzymes produced by bacteria that break open the β-lactam ring, inactivating the β-lactam antibiotic .
Mode of Action
This compound acts as an inhibitor of β-lactamases . It interacts with these enzymes, preventing them from hydrolyzing and inactivating β-lactam antibiotics . This allows the β-lactam antibiotics to remain intact and continue to inhibit bacterial transpeptidases, also known as penicillin-binding proteins (PBPs), which are involved in peptidoglycan synthesis .
Biochemical Pathways
The action of β-lactamase-IN-1 affects the biochemical pathway of peptidoglycan synthesis . By inhibiting β-lactamases, β-lactam antibiotics can continue to bind to PBPs and disrupt peptidoglycan synthesis, inhibiting bacterial growth and leading to bacterial lysis .
Pharmacokinetics
The pharmacokinetics of β-lactamase inhibitors like this compound is complex and involves a two-component pharmacodynamic system . The β-lactamase inhibitor inactivates the bacterial β-lactamase enzyme, freeing the companion β-lactam to act against its penicillin-binding protein target . The pharmacokinetic/pharmacodynamic index that describes the efficacy of β-lactamase inhibitors is variable among inhibitors .
Result of Action
The result of the action of this compound is the prevention of β-lactam antibiotic inactivation . This allows the β-lactam antibiotics to inhibit bacterial growth and lead to bacterial lysis . Thus, the use of this compound can help overcome antibiotic resistance in bacteria that produce β-lactamases .
Action Environment
The action of this compound can be influenced by various environmental factors. For instance, the presence of β-lactamases in the environment has been known since 1940, suggesting that these enzymes have their origin more than 2 billion years ago . Over the past eight decades, β-lactamases have come into focus as factors of antibiotic resistance . The effectiveness of this compound can also be influenced by the genetic plasticity of bacteria, which can contribute to their ability to resist a variety of disinfectants and antibiotics .
Preparation Methods
Synthetic Routes and Reaction Conditions
The synthesis of beta-lactamase-IN-1 typically involves the formation of a beta-lactam ring, which is a four-membered lactam. The synthetic route may include the following steps:
Formation of the beta-lactam ring: This can be achieved through cyclization reactions involving amino acids or peptides.
Functionalization: The beta-lactam ring is then functionalized with various substituents to enhance its inhibitory activity against beta-lactamase enzymes
Industrial Production Methods
Industrial production of this compound involves large-scale synthesis using optimized reaction conditions to ensure high yield and purity. This may include:
Batch processing: Large reactors are used to carry out the synthesis in batches.
Continuous processing: A continuous flow of reactants is maintained to produce the compound in a steady state
Chemical Reactions Analysis
Types of Reactions
Beta-lactamase-IN-1 undergoes various chemical reactions, including:
Hydrolysis: The beta-lactam ring can be hydrolyzed by beta-lactamase enzymes, leading to the formation of inactive products.
Oxidation and Reduction: These reactions can modify the functional groups attached to the beta-lactam ring
Common Reagents and Conditions
Hydrolysis: Water or aqueous solutions are used under mild conditions.
Oxidation: Oxidizing agents such as hydrogen peroxide or potassium permanganate.
Reduction: Reducing agents like sodium borohydride or lithium aluminum hydride
Major Products
The major products formed from these reactions include various derivatives of the beta-lactam ring, which may have different levels of inhibitory activity against beta-lactamase enzymes .
Scientific Research Applications
Beta-lactamase-IN-1 has a wide range of applications in scientific research, including:
Chemistry: Used as a tool to study the mechanisms of beta-lactamase enzymes and develop new inhibitors.
Biology: Helps in understanding bacterial resistance mechanisms and the role of beta-lactamase enzymes in bacterial physiology.
Medicine: Used in combination with beta-lactam antibiotics to treat infections caused by resistant bacterial strains.
Industry: Employed in the development of new antibacterial agents and diagnostic tools
Comparison with Similar Compounds
Beta-lactamase-IN-1 is unique in its high specificity and potency against beta-lactamase enzymes. Similar compounds include:
Clavulanic acid: Another beta-lactamase inhibitor, but with a different structure and mechanism of action.
Sulbactam: A beta-lactamase inhibitor that is often used in combination with beta-lactam antibiotics.
Tazobactam: Similar to sulbactam, it is used to enhance the efficacy of beta-lactam antibiotics
This compound stands out due to its ability to inhibit a broader range of beta-lactamase enzymes and its potential for use in various therapeutic applications.
Properties
IUPAC Name |
4-(1,3-dihydroxypropan-2-yl)-6-methoxypyrido[2,3-b]pyrazin-3-one | |
---|---|---|
Source | PubChem | |
URL | https://pubchem.ncbi.nlm.nih.gov | |
Description | Data deposited in or computed by PubChem | |
InChI |
InChI=1S/C11H13N3O4/c1-18-9-3-2-8-11(13-9)14(7(5-15)6-16)10(17)4-12-8/h2-4,7,15-16H,5-6H2,1H3 | |
Source | PubChem | |
URL | https://pubchem.ncbi.nlm.nih.gov | |
Description | Data deposited in or computed by PubChem | |
InChI Key |
VOAJCTMMHSBQGS-UHFFFAOYSA-N | |
Source | PubChem | |
URL | https://pubchem.ncbi.nlm.nih.gov | |
Description | Data deposited in or computed by PubChem | |
Canonical SMILES |
COC1=NC2=C(C=C1)N=CC(=O)N2C(CO)CO | |
Source | PubChem | |
URL | https://pubchem.ncbi.nlm.nih.gov | |
Description | Data deposited in or computed by PubChem | |
Molecular Formula |
C11H13N3O4 | |
Source | PubChem | |
URL | https://pubchem.ncbi.nlm.nih.gov | |
Description | Data deposited in or computed by PubChem | |
DSSTOX Substance ID |
DTXSID501141541 | |
Record name | 4-[2-Hydroxy-1-(hydroxymethyl)ethyl]-6-methoxypyrido[2,3-b]pyrazin-3(4H)-one | |
Source | EPA DSSTox | |
URL | https://comptox.epa.gov/dashboard/DTXSID501141541 | |
Description | DSSTox provides a high quality public chemistry resource for supporting improved predictive toxicology. | |
Molecular Weight |
251.24 g/mol | |
Source | PubChem | |
URL | https://pubchem.ncbi.nlm.nih.gov | |
Description | Data deposited in or computed by PubChem | |
CAS No. |
1075237-97-6 | |
Record name | 4-[2-Hydroxy-1-(hydroxymethyl)ethyl]-6-methoxypyrido[2,3-b]pyrazin-3(4H)-one | |
Source | CAS Common Chemistry | |
URL | https://commonchemistry.cas.org/detail?cas_rn=1075237-97-6 | |
Description | CAS Common Chemistry is an open community resource for accessing chemical information. Nearly 500,000 chemical substances from CAS REGISTRY cover areas of community interest, including common and frequently regulated chemicals, and those relevant to high school and undergraduate chemistry classes. This chemical information, curated by our expert scientists, is provided in alignment with our mission as a division of the American Chemical Society. | |
Explanation | The data from CAS Common Chemistry is provided under a CC-BY-NC 4.0 license, unless otherwise stated. | |
Record name | 4-[2-Hydroxy-1-(hydroxymethyl)ethyl]-6-methoxypyrido[2,3-b]pyrazin-3(4H)-one | |
Source | EPA DSSTox | |
URL | https://comptox.epa.gov/dashboard/DTXSID501141541 | |
Description | DSSTox provides a high quality public chemistry resource for supporting improved predictive toxicology. | |
Synthesis routes and methods
Procedure details
Retrosynthesis Analysis
AI-Powered Synthesis Planning: Our tool employs the Template_relevance Pistachio, Template_relevance Bkms_metabolic, Template_relevance Pistachio_ringbreaker, Template_relevance Reaxys, Template_relevance Reaxys_biocatalysis model, leveraging a vast database of chemical reactions to predict feasible synthetic routes.
One-Step Synthesis Focus: Specifically designed for one-step synthesis, it provides concise and direct routes for your target compounds, streamlining the synthesis process.
Accurate Predictions: Utilizing the extensive PISTACHIO, BKMS_METABOLIC, PISTACHIO_RINGBREAKER, REAXYS, REAXYS_BIOCATALYSIS database, our tool offers high-accuracy predictions, reflecting the latest in chemical research and data.
Strategy Settings
Precursor scoring | Relevance Heuristic |
---|---|
Min. plausibility | 0.01 |
Model | Template_relevance |
Template Set | Pistachio/Bkms_metabolic/Pistachio_ringbreaker/Reaxys/Reaxys_biocatalysis |
Top-N result to add to graph | 6 |
Feasible Synthetic Routes
Q1: What are extended-spectrum beta-lactamases (ESBLs)?
A1: ESBLs are enzymes produced by some bacteria that make them resistant to commonly used antibiotics like penicillins and cephalosporins. These enzymes break down the structure of these antibiotics, rendering them ineffective. []
Q2: What are the most common types of ESBLs?
A2: The most common ESBLs are derived from TEM-1, SHV-1, CTX-M, and OXA-type enzymes. [, ]
Q3: Can resistance to beta-lactam antibiotics arise from mechanisms other than beta-lactamase production?
A3: Yes, resistance can also occur due to mutations in porin proteins, which are found in the outer membrane of some bacteria. These mutations can prevent antibiotics from entering the bacterial cell. []
Q4: Are metallo-beta-lactamases (MBLs) a significant concern?
A4: Yes, MBLs are a major concern because they can hydrolyze a broad spectrum of beta-lactam antibiotics, including carbapenems, which are often considered "last-resort" antibiotics. [, ]
Q5: What is the significance of plasmids in antibiotic resistance?
A5: Plasmids are small, circular DNA molecules that can be transferred between bacteria. They often carry genes that encode for antibiotic resistance, making their spread a serious concern in the fight against antibiotic resistance. [, , ]
Disclaimer and Information on In-Vitro Research Products
Please be aware that all articles and product information presented on BenchChem are intended solely for informational purposes. The products available for purchase on BenchChem are specifically designed for in-vitro studies, which are conducted outside of living organisms. In-vitro studies, derived from the Latin term "in glass," involve experiments performed in controlled laboratory settings using cells or tissues. It is important to note that these products are not categorized as medicines or drugs, and they have not received approval from the FDA for the prevention, treatment, or cure of any medical condition, ailment, or disease. We must emphasize that any form of bodily introduction of these products into humans or animals is strictly prohibited by law. It is essential to adhere to these guidelines to ensure compliance with legal and ethical standards in research and experimentation.