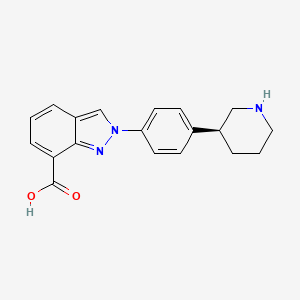
Niraparib metabolite M1
Overview
Description
Niraparib metabolite M1, also known as niraparib carboxylic acid, is a primary metabolite of niraparib. Niraparib is an orally active poly (ADP-Ribose) polymerase inhibitor used in the treatment of recurrent epithelial ovarian, fallopian tube, or primary peritoneal cancer. This compound is formed through the hydrolysis of niraparib and plays a crucial role in the drug’s metabolic pathway .
Mechanism of Action
Target of Action
Niraparib metabolite M1 is a major inactive metabolite of Niraparib . Niraparib is an orally active poly (ADP-ribose) polymerase (PARP) inhibitor . It is selective towards PARP-1 and PARP-2 , which are enzymes responsible for DNA repair .
Mode of Action
By blocking the enzymes responsible for DNA repair, Niraparib induces cytotoxicity in cancer cells . This action leads to the accumulation of DNA damage and ultimately cell death, particularly in cancer cells that already have deficiencies in DNA repair mechanisms .
Biochemical Pathways
Niraparib is primarily metabolized by carboxylesterases (CEs) to form M1 . The M1 metabolite can subsequently undergo glucuronidation mediated by UDP-glucuronosyltransferases (UGTs) to form the M10 metabolite . In a mass balance study, M1 and M10 were the major circulating metabolites .
Pharmacokinetics
Both renal and hepatic pathways are comparably involved in the excretion of Niraparib and its metabolites . The elimination of radioactivity was slow, with a half-life in plasma on average 92.5 hours . Oral absorption of Niraparib was rapid, with Niraparib concentrations peaking at 2.49 hours, and reaching a mean maximum concentration of 540 ng/mL .
Result of Action
The action of Niraparib leads to the accumulation of DNA damage and ultimately cell death, particularly in cancer cells that already have deficiencies in DNA repair mechanisms . This results in the cytotoxicity of cancer cells .
Action Environment
The action, efficacy, and stability of Niraparib and its metabolites can be influenced by various environmental factors. For instance, the pharmacokinetics of Niraparib can be affected by the patient’s renal and hepatic function
Biochemical Analysis
Biochemical Properties
Niraparib Metabolite M1 is formed through the action of carboxylesterases (CEs) on Niraparib . This metabolite can subsequently undergo glucuronidation mediated by UDP-glucuronosyltransferases (UGTs) to form the M10 metabolite . The oxidative pathway is minimal in the metabolism of Niraparib .
Cellular Effects
As an inactive metabolite of Niraparib, this compound does not directly influence cell function, cell signaling pathways, gene expression, or cellular metabolism . Its formation is a key part of the metabolism and elimination of Niraparib, a drug that has significant cellular effects due to its inhibition of PARP enzymes .
Molecular Mechanism
The molecular mechanism of this compound primarily involves its formation and further metabolism. Niraparib is metabolized by carboxylesterases to form this compound, which is then subject to glucuronidation .
Temporal Effects in Laboratory Settings
The formation of this compound from Niraparib is a part of the drug’s metabolism and elimination process. In a study, the elimination of radioactivity associated with Niraparib was slow, with a half-life in plasma averaging 92.5 hours . This suggests that the formation and elimination of this compound may also occur over a relatively long timescale.
Metabolic Pathways
This compound is part of the metabolic pathway of Niraparib. Niraparib is metabolized by carboxylesterases to form this compound, which can then undergo glucuronidation .
Preparation Methods
Synthetic Routes and Reaction Conditions: Niraparib metabolite M1 is synthesized through the hydrolysis of niraparib. The process involves the cleavage of the amide bond in niraparib, resulting in the formation of niraparib carboxylic acid. This reaction can be catalyzed by carboxylesterases, which are enzymes that facilitate the hydrolysis of ester and amide bonds .
Industrial Production Methods: The industrial production of this compound involves the large-scale synthesis of niraparib followed by its enzymatic hydrolysis. The reaction conditions are optimized to ensure high yield and purity of the metabolite. The process typically involves the use of bioreactors where the enzymatic reaction is carried out under controlled conditions of temperature, pH, and enzyme concentration .
Chemical Reactions Analysis
Types of Reactions: Niraparib metabolite M1 primarily undergoes hydrolytic and conjugative metabolic conversions. The oxidative pathway is minimal in the metabolism of niraparib .
Common Reagents and Conditions:
Hydrolysis: Catalyzed by carboxylesterases under physiological conditions.
Conjugation: Involves the addition of glucuronic acid to form the glucuronide of M1. .
Major Products:
Hydrolysis Product: Niraparib carboxylic acid (M1).
Conjugation Product: Glucuronide of M1.
Scientific Research Applications
Niraparib metabolite M1 has several scientific research applications, particularly in the fields of chemistry, biology, medicine, and industry:
Comparison with Similar Compounds
Niraparib metabolite M1 can be compared with other metabolites of poly (ADP-Ribose) polymerase inhibitors, such as:
Olaparib Metabolite M1: Similar to this compound, olaparib metabolite M1 is formed through the hydrolysis of olaparib.
Rucaparib Metabolite M1: Another poly (ADP-Ribose) polymerase inhibitor metabolite formed through hydrolysis.
Uniqueness: this compound is unique in its formation and subsequent metabolic conversions. The minimal involvement of the oxidative pathway distinguishes it from other metabolites that may undergo significant oxidative metabolism .
Properties
IUPAC Name |
2-[4-[(3S)-piperidin-3-yl]phenyl]indazole-7-carboxylic acid | |
---|---|---|
Source | PubChem | |
URL | https://pubchem.ncbi.nlm.nih.gov | |
Description | Data deposited in or computed by PubChem | |
InChI |
InChI=1S/C19H19N3O2/c23-19(24)17-5-1-3-15-12-22(21-18(15)17)16-8-6-13(7-9-16)14-4-2-10-20-11-14/h1,3,5-9,12,14,20H,2,4,10-11H2,(H,23,24)/t14-/m1/s1 | |
Source | PubChem | |
URL | https://pubchem.ncbi.nlm.nih.gov | |
Description | Data deposited in or computed by PubChem | |
InChI Key |
HDQHGRLURKGIFL-CQSZACIVSA-N | |
Source | PubChem | |
URL | https://pubchem.ncbi.nlm.nih.gov | |
Description | Data deposited in or computed by PubChem | |
Canonical SMILES |
C1CC(CNC1)C2=CC=C(C=C2)N3C=C4C=CC=C(C4=N3)C(=O)O | |
Source | PubChem | |
URL | https://pubchem.ncbi.nlm.nih.gov | |
Description | Data deposited in or computed by PubChem | |
Isomeric SMILES |
C1C[C@H](CNC1)C2=CC=C(C=C2)N3C=C4C=CC=C(C4=N3)C(=O)O | |
Source | PubChem | |
URL | https://pubchem.ncbi.nlm.nih.gov | |
Description | Data deposited in or computed by PubChem | |
Molecular Formula |
C19H19N3O2 | |
Source | PubChem | |
URL | https://pubchem.ncbi.nlm.nih.gov | |
Description | Data deposited in or computed by PubChem | |
Molecular Weight |
321.4 g/mol | |
Source | PubChem | |
URL | https://pubchem.ncbi.nlm.nih.gov | |
Description | Data deposited in or computed by PubChem | |
CAS No. |
1476777-06-6 | |
Record name | 2H-Indazole-7-carboxylic acid, 2-(4-(3S)-3-piperidinylphenyl)- | |
Source | ChemIDplus | |
URL | https://pubchem.ncbi.nlm.nih.gov/substance/?source=chemidplus&sourceid=1476777066 | |
Description | ChemIDplus is a free, web search system that provides access to the structure and nomenclature authority files used for the identification of chemical substances cited in National Library of Medicine (NLM) databases, including the TOXNET system. | |
Record name | 2H-INDAZOLE-7-CARBOXYLIC ACID, 2-(4-(3S)-3-PIPERIDINYLPHENYL)- | |
Source | FDA Global Substance Registration System (GSRS) | |
URL | https://gsrs.ncats.nih.gov/ginas/app/beta/substances/UXM824C9GS | |
Description | The FDA Global Substance Registration System (GSRS) enables the efficient and accurate exchange of information on what substances are in regulated products. Instead of relying on names, which vary across regulatory domains, countries, and regions, the GSRS knowledge base makes it possible for substances to be defined by standardized, scientific descriptions. | |
Explanation | Unless otherwise noted, the contents of the FDA website (www.fda.gov), both text and graphics, are not copyrighted. They are in the public domain and may be republished, reprinted and otherwise used freely by anyone without the need to obtain permission from FDA. Credit to the U.S. Food and Drug Administration as the source is appreciated but not required. | |
Disclaimer and Information on In-Vitro Research Products
Please be aware that all articles and product information presented on BenchChem are intended solely for informational purposes. The products available for purchase on BenchChem are specifically designed for in-vitro studies, which are conducted outside of living organisms. In-vitro studies, derived from the Latin term "in glass," involve experiments performed in controlled laboratory settings using cells or tissues. It is important to note that these products are not categorized as medicines or drugs, and they have not received approval from the FDA for the prevention, treatment, or cure of any medical condition, ailment, or disease. We must emphasize that any form of bodily introduction of these products into humans or animals is strictly prohibited by law. It is essential to adhere to these guidelines to ensure compliance with legal and ethical standards in research and experimentation.