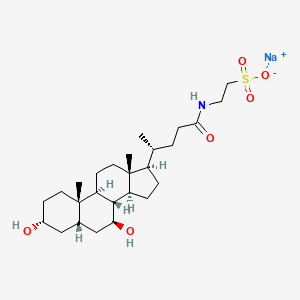
Sodium tauroursodeoxycholate
- Click on QUICK INQUIRY to receive a quote from our team of experts.
- With the quality product at a COMPETITIVE price, you can focus more on your research.
Overview
Description
Sodium tauroursodeoxycholate (TUDCA) is a hydrophilic, taurine-conjugated bile acid derivative of ursodeoxycholic acid (UDCA). It is a naturally occurring tertiary bile acid with notable cytoprotective, anti-apoptotic, and anti-inflammatory properties . Structurally, TUDCA consists of a steroid nucleus with hydroxyl groups at positions 3α, 7β, and a taurine moiety conjugated to the side chain, enhancing its solubility and bioavailability compared to unconjugated bile acids .
TUDCA is clinically used for treating cholestatic liver diseases (e.g., primary biliary cholangitis), gallstones, and metabolic disorders such as diabetes and neurodegenerative conditions . Its mechanism of action includes reducing endoplasmic reticulum (ER) stress, stabilizing mitochondrial membranes, and modulating bile acid enterohepatic circulation via interactions with transporters like the human apical sodium-dependent bile acid transporter (hASBT) .
Preparation Methods
Synthetic Routes and Reaction Conditions: The preparation of sodium tauroursodeoxycholate typically involves the reaction of ursodeoxycholic acid with taurine. One common method includes the formation of a mixed anhydride of ursodeoxycholic acid with an alkyl chloroformate, followed by reaction with an aqueous solution of sodium taurinate . The reaction conditions often involve selective precipitation to remove impurities and achieve a high-purity product .
Industrial Production Methods: Industrial production of this compound follows similar synthetic routes but on a larger scale. The process involves careful control of reaction conditions to ensure consistent quality and yield. The use of advanced purification techniques, such as crystallization and filtration, is common to obtain the final product suitable for pharmaceutical applications .
Chemical Reactions Analysis
Types of Reactions: Sodium tauroursodeoxycholate undergoes various chemical reactions, including:
Oxidation: This reaction can lead to the formation of oxidized bile acid derivatives.
Reduction: Reduction reactions can modify the hydroxyl groups present in the compound.
Substitution: Substitution reactions often involve the replacement of functional groups with other chemical entities.
Common Reagents and Conditions:
Oxidizing Agents: Hydrogen peroxide, potassium permanganate.
Reducing Agents: Sodium borohydride, lithium aluminum hydride.
Substitution Reagents: Halogenating agents, sulfonating agents.
Major Products Formed: The major products formed from these reactions depend on the specific reagents and conditions used. For example, oxidation can produce more hydrophilic bile acid derivatives, while reduction can lead to the formation of less hydrophilic compounds .
Scientific Research Applications
Hepatobiliary Disorders
- Gallstone Prevention and Treatment : TUDCA is primarily used in Europe to prevent and treat gallstones. It works by reducing cholesterol levels and increasing bile acid content in the gallbladder, thereby preventing cholesterol gallstone formation .
- Cholestatic Liver Diseases : Approved for use in primary biliary cholangitis, TUDCA helps improve liver function by alleviating cholestasis and reducing liver inflammation .
Neurodegenerative Diseases
TUDCA has shown promise in treating several neurodegenerative diseases due to its cytoprotective properties:
- Amyotrophic Lateral Sclerosis (ALS) : A recent study indicated that patients treated with TUDCA had improved overall survival rates compared to those who did not receive treatment. The median survival for TUDCA-treated patients was 49.6 months versus 36.2 months for controls .
- Alzheimer's Disease : TUDCA may mitigate endoplasmic reticulum stress, which is implicated in neuronal death associated with Alzheimer's disease .
- Parkinson's Disease : Research suggests that TUDCA can stabilize mitochondrial membranes and enhance oxidative defenses, potentially offering neuroprotection against Parkinson's disease .
Metabolic Disorders
TUDCA has been investigated for its role in metabolic diseases such as obesity and diabetes:
- Obesity : Studies indicate that TUDCA can suppress adipogenesis and promote angiogenesis in adipose-derived mesenchymal stem cells, suggesting a role in weight management .
- Diabetes : Its cytoprotective effects extend to insulin-producing pancreatic cells, where TUDCA may help preserve cell function under stress conditions .
Ocular Disorders
Recent animal studies have shown that TUDCA can protect photoreceptors from cell death following retinal detachment, indicating potential applications in treating retinal diseases such as age-related macular degeneration .
Case Study 1: ALS Treatment with TUDCA
In a retrospective analysis involving 86 ALS patients treated with TUDCA, results showed that higher dosages (≥1000 mg/day) were associated with significantly reduced mortality risk (HR 0.56) compared to controls . The treatment was generally well-tolerated with minimal side effects.
Case Study 2: Ocular Protection
A study on rats demonstrated that systemic administration of TUDCA significantly reduced photoreceptor loss after induced retinal detachment, highlighting its potential as a protective agent in ocular health .
Data Summary Table
Application Area | Condition | Mechanism of Action | Key Findings |
---|---|---|---|
Hepatobiliary Disorders | Gallstones | Reduces cholesterol levels | Prevents formation of cholesterol gallstones |
Neurodegenerative Diseases | ALS | Anti-apoptotic, ER stress reduction | Improved overall survival rates |
Alzheimer's | Stabilizes mitochondrial membranes | Mitigates neuronal death | |
Parkinson's | Enhances oxidative defenses | Provides neuroprotection | |
Metabolic Disorders | Obesity | Suppresses adipogenesis | Promotes weight management |
Diabetes | Preserves pancreatic cell function | Improves insulin secretion under stress | |
Ocular Disorders | Retinal Detachment | Protects photoreceptors | Reduces photoreceptor loss |
Mechanism of Action
The mechanism of action of sodium tauroursodeoxycholate involves several molecular pathways:
Anti-apoptotic Effects: It inhibits the intrinsic mitochondrial apoptotic pathway by reducing reactive oxygen species (ROS) and inactivating BAX, thereby decreasing cytochrome c release.
ER Stress Reduction: It reduces endoplasmic reticulum (ER) stress by decreasing caspase 12 activity and calcium efflux from the ER.
Anti-inflammatory Action: It exerts anti-inflammatory effects by modulating immune responses and reducing oxidative stress.
Comparison with Similar Compounds
Structural and Physicochemical Properties
TUDCA is distinguished from other bile acids by its taurine conjugation and hydroxylation pattern. Key comparisons include:
Notes:
- Hydrophobicity indices (standardized to taurocholate = 0) influence membrane interactions and toxicity. TUDCA’s lower index enhances biocompatibility .
- Taurine conjugation increases solubility and reduces detergent-like membrane disruption compared to glycine-conjugated or unconjugated bile acids .
Chaperone Activity and ER Stress Mitigation
TUDCA outperforms UDCA and other bile acids in mitigating ER stress. In human lung epithelial cells, TUDCA (10–50 μM) prevented paraquat-induced apoptosis by suppressing ER stress pathways, while UDCA required higher concentrations for similar effects .
Transporter Interactions
TUDCA exhibits moderate inhibitory potency against hASBT (Ki ~0.5 μM), comparable to lithocholate conjugates but significantly stronger than UDCA (Ki ~20 μM) . This suggests TUDCA may modulate bile acid reabsorption more effectively in cholestasis .
Cytoprotection and Toxicity
In chronic fatigue syndrome models, chronic exposure to TUDCA (10 mg/mL in ethanol) showed chemopreventative effects without toxicity, whereas ursodeoxycholate caused dose-dependent cytotoxicity . TUDCA’s low toxicity is attributed to its hydrophilic nature, which minimizes membrane disruption .
Metabolic and Pharmacokinetic Profiles
Absorption and Metabolism
TUDCA is better absorbed than UDCA in humans, with reduced conversion to toxic metabolites like lithocholic acid. In patients with primary biliary cholangitis, TUDCA administration resulted in higher biliary enrichment (32.6% vs. 29.2% for UDCA) and lower fecal excretion of hydrophobic metabolites .
Species-Specific Effects
In rats, TUDCA infusion enhanced taurocholate excretion and prevented cholestasis more effectively than taurodehydrocholate . However, taurocholate itself showed stronger anti-cholestatic effects against taurolithocholate, highlighting context-dependent efficacy .
Key Research Findings
- ER Stress Inhibition : TUDCA (10 μM) suppressed HIV-1 replication in CD4+ T cells by inhibiting ER stress pathways, a mechanism absent in UDCA-treated cells .
- Metabolomic Biomarker : Plasma TUDCA levels correlated with myalgic encephalomyelitis/chronic fatigue syndrome (ME/CFS) severity, unlike other bile acids .
- Hepatoprotection : In cholestatic rats, TUDCA (360 μmol/kg) restored bile flow and reduced liver enzyme elevation, outperforming UDCA .
Limitations and Contradictions
- While TUDCA shows superior safety in most studies, its efficacy in taurolithocholate-induced cholestasis was weaker than taurocholate .
Biological Activity
Sodium tauroursodeoxycholate (TUDCA) is a bile acid derivative known for its diverse biological activities, particularly in the context of cellular protection and therapeutic applications. This article provides a comprehensive overview of the biological activity of TUDCA, focusing on its mechanisms of action, clinical implications, and relevant research findings.
TUDCA is a taurine-conjugated form of ursodeoxycholic acid (UDCA), which has been utilized in traditional medicine for centuries. Its chemical structure allows it to function as a chemical chaperone , alleviating cellular stress by stabilizing proteins and promoting proper folding. The primary mechanisms through which TUDCA exerts its biological effects include:
- Endoplasmic Reticulum (ER) Stress Alleviation : TUDCA reduces ER stress by modulating the unfolded protein response (UPR), which is crucial in maintaining cellular homeostasis during stress conditions .
- Cytoprotection : TUDCA exhibits protective effects against various forms of cell death, including apoptosis induced by toxins like paraquat. It has been shown to suppress ER stress responses in human lung epithelial cells, thereby preventing cell death .
- Choleretic Activity : TUDCA enhances bile flow and reduces cholestasis by stimulating the exocytosis of key transport proteins in hepatocytes .
In Vitro Studies
- Cholangiocarcinoma Cells : In studies involving Mz-ChA-1 cholangiocarcinoma cells, TUDCA inhibited cell growth through calcium-dependent pathways, highlighting its potential as an anti-cancer agent .
- Hepatocyte Protection : In isolated rat hepatocytes, TUDCA inhibited bile acid-induced apoptosis via β1-integrin-mediated cAMP formation, demonstrating its protective role against liver injury .
In Vivo Studies
- Cholestasis Model : In phalloidin-induced cholestasis models in rats, TUDCA significantly improved bile flow and reduced serum levels of liver enzymes (alkaline phosphatase, leucine aminopeptidase) when administered intravenously at a dose of 360 μmol/kg .
- Neuroprotective Effects : Research indicates that TUDCA may have neuroprotective effects in models of neurodegenerative diseases by mitigating ER stress and promoting neuronal survival .
Clinical Applications
TUDCA has gained attention for its therapeutic potential beyond hepatobiliary disorders:
- Diabetes and Obesity : Recent studies suggest that TUDCA may improve insulin sensitivity and reduce fat accumulation, indicating a role in metabolic disorders .
- Neurodegenerative Diseases : Its ability to alleviate ER stress positions TUDCA as a candidate for treating conditions like Alzheimer's disease and amyotrophic lateral sclerosis (ALS) .
Data Summary Table
Q & A
Basic Research Questions
Q. What are the standard experimental protocols for identifying and quantifying sodium tauroursodeoxycholate (TUDC) in biological samples?
TUDC identification typically employs infrared (IR) spectroscopy (absorption peaks at ~2930 cm⁻¹, 1645 cm⁻¹, and 1049 cm⁻¹) and thin-layer chromatography (TLC) with methanol as a solvent . For quantification, high-performance liquid chromatography coupled with tandem mass spectrometry (HPLC-MS/MS) is widely used, as demonstrated in studies analyzing bile acids in complex matrices like bear bile powder . Ensure sample purity ≥98% (verified via TLC spot intensity comparisons) and account for hydration states (e.g., molecular weight differences between anhydrous and hydrated forms) .
Q. How are in vitro and in vivo models optimized to study TUDC's hepatoprotective effects?
- In vitro : Use primary rat hepatocytes or human cell lines (e.g., A549 lung epithelial cells) treated with toxins like paraquat or glycochenodeoxycholate (GCDC). TUDC concentrations of 100–200 µM are common, with endpoints including apoptosis markers (e.g., caspase activation) and ER stress proteins (e.g., BiP/GRP78) .
- In vivo : Rat models of cholestasis (e.g., phalloidin-induced) or liver injury are standard. Administer TUDC intravenously at 360 µmol/kg to observe bile flow restoration and reductions in serum alkaline phosphatase .
Advanced Research Questions
Q. What molecular mechanisms underlie TUDC's inhibition of endoplasmic reticulum (ER) stress, and how do these pathways intersect with apoptosis regulation?
TUDC acts as a chemical chaperone, stabilizing misfolded proteins and suppressing the unfolded protein response (UPR). Key mechanisms include:
- IRE1α-XBP1 pathway inhibition : Reduces pro-apoptotic CHOP expression .
- ERK1/2 phosphorylation : Activates survival signaling, countering GCDC-induced apoptosis in hepatocytes .
- Calcium homeostasis : Modulates Ca²⁺-dependent PKC and MAPK pathways to inhibit bile acid toxicity . Contradictions arise in cell-type-specific responses; for example, TUDC upregulates anti-apoptotic p53 targets in perfused livers but shows variable efficacy in cancer cell lines .
Q. How can researchers reconcile contradictory data on TUDC's efficacy across disease models, such as diabetic retinopathy vs. cholestasis?
Discrepancies often stem from:
- Dose-dependent effects : Lower doses (e.g., 100 µM) enhance cell proliferation in neural stem cells, while higher doses (200 µM) suppress ER stress in epithelial cells .
- Microbiome interactions : Gut microbiota modify TUDC's bioavailability and metabolite profile, impacting outcomes in metabolic diseases like diabetes .
- Model limitations : Immortalized cell lines may lack physiological relevance compared to organoid or induced pluripotent stem cell (iPSC)-derived models .
Q. What innovative methodologies are emerging for studying TUDC's pleiotropic effects in multi-organ systems?
- Multi-omics integration : Combine transcriptomics (e.g., RNA-seq of ER stress genes) with metabolomics (bile acid profiling) to map TUDC's systemic impacts .
- Microphysiological systems (MPS) : Liver-on-a-chip platforms enable real-time analysis of TUDC's modulation of bile acid transport and toxicity .
- CRISPR screening : Identify novel TUDC targets (e.g., WDR45 mutations linked to autophagy dysregulation) using genome-wide knockout libraries .
Q. Methodological Considerations
Q. What are critical factors in designing reproducible studies on TUDC's therapeutic potential?
- Solubility and formulation : TUDC is freely soluble in water (≥8.42 mg/mL) and methanol but sparingly soluble in ethanol. Use sterile saline for in vivo studies to avoid precipitation .
- Controls : Include bile acid analogs (e.g., taurochenodeoxycholate) to isolate TUDC-specific effects .
- Endpoint validation : Use orthogonal assays (e.g., Western blot for ER stress markers and flow cytometry for apoptosis) to confirm findings .
Q. How can researchers address variability in TUDC's reported IC₅₀ values across cell lines?
Variability often reflects differences in:
- Cell permeability : Hydrophilic TUDC requires optimized delivery (e.g., dimethyl sulfoxide (DMSO) carriers for hydrophobic matrices) .
- Receptor expression levels : TGR5 receptor density influences TUDC's efficacy in metabolic regulation . Standardize assays using reference compounds (e.g., 4-phenylbutyrate for ER stress inhibition) and report cell passage numbers to enhance comparability .
Properties
CAS No. |
35807-85-3 |
---|---|
Molecular Formula |
C26H44NNaO6S |
Molecular Weight |
521.7 g/mol |
IUPAC Name |
sodium;2-[4-[(10S,13R)-3,7-dihydroxy-10,13-dimethyl-2,3,4,5,6,7,8,9,11,12,14,15,16,17-tetradecahydro-1H-cyclopenta[a]phenanthren-17-yl]pentanoylamino]ethanesulfonate |
InChI |
InChI=1S/C26H45NO6S.Na/c1-16(4-7-23(30)27-12-13-34(31,32)33)19-5-6-20-24-21(9-11-26(19,20)3)25(2)10-8-18(28)14-17(25)15-22(24)29;/h16-22,24,28-29H,4-15H2,1-3H3,(H,27,30)(H,31,32,33);/q;+1/p-1/t16?,17?,18?,19?,20?,21?,22?,24?,25-,26+;/m0./s1 |
InChI Key |
IYPNVUSIMGAJFC-ZPTIPWSTSA-M |
SMILES |
CC(CCC(=O)NCCS(=O)(=O)[O-])C1CCC2C1(CCC3C2C(CC4C3(CCC(C4)O)C)O)C.[Na+] |
Isomeric SMILES |
CC(CCC(=O)NCCS(=O)(=O)[O-])C1CCC2[C@@]1(CCC3C2C(CC4[C@@]3(CCC(C4)O)C)O)C.[Na+] |
Canonical SMILES |
CC(CCC(=O)NCCS(=O)(=O)[O-])C1CCC2C1(CCC3C2C(CC4C3(CCC(C4)O)C)O)C.[Na+] |
Synonyms |
NA |
Origin of Product |
United States |
Disclaimer and Information on In-Vitro Research Products
Please be aware that all articles and product information presented on BenchChem are intended solely for informational purposes. The products available for purchase on BenchChem are specifically designed for in-vitro studies, which are conducted outside of living organisms. In-vitro studies, derived from the Latin term "in glass," involve experiments performed in controlled laboratory settings using cells or tissues. It is important to note that these products are not categorized as medicines or drugs, and they have not received approval from the FDA for the prevention, treatment, or cure of any medical condition, ailment, or disease. We must emphasize that any form of bodily introduction of these products into humans or animals is strictly prohibited by law. It is essential to adhere to these guidelines to ensure compliance with legal and ethical standards in research and experimentation.