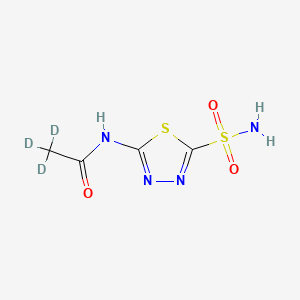
Acetazolamide-d3
Overview
Description
Acetazolamide-d3 is a deuterated form of acetazolamide, a carbonic anhydrase inhibitor. It is commonly used in scientific research as an internal standard in mass spectrometry due to its stable isotopic labeling. Acetazolamide itself is used to treat conditions such as glaucoma, epilepsy, mountain sickness, periodic paralysis, central sleep apnea, and idiopathic intracranial hypertension .
Mechanism of Action
Target of Action
Acetazolamide-d3, also known as Acetazolamide, primarily targets the enzyme carbonic anhydrase . This enzyme plays a crucial role in maintaining the balance of carbon dioxide and water in the body .
Mode of Action
This compound acts by reversibly inhibiting the carbonic anhydrase enzyme . This inhibition results in a reduction of hydrogen ion secretion at the renal tubule and an increased renal excretion of sodium, potassium, bicarbonate, and water . In vitro studies have also found that this compound acidifies the cytosol and activates kinases that subsequently force synaptic recycling mechanisms, hence modulating synaptic performance .
Biochemical Pathways
The inhibition of carbonic anhydrase by this compound affects several biochemical pathways. It leads to a decrease in the formation of hydrogen ions and bicarbonate from carbon dioxide and water . This action has a significant impact on the body’s pH balance and fluid regulation . Furthermore, it has been suggested that more contemporary studies have focused on cellular membrane ion/water channel activity .
Pharmacokinetics
After oral ingestion, this compound is rapidly absorbed with a Tmax of 2–4 hours and a bioavailability of >90% . Its volume of distribution is 0.3 L/kg, and plasma protein binding is 90–95% . The plasma elimination half-life values in adults are 10–15 hours .
Action Environment
The action, efficacy, and stability of this compound can be influenced by various environmental factors. For instance, the acid-base status of the patient may alter the distribution of this compound in the cerebrospinal fluid (CSF) and brain . Furthermore, the drug’s efficacy in the context of acute mountain sickness (AMS) is likely due to a multitude of effects, including metabolic acidosis resulting from renal carbonic anhydrase inhibition, improvements in ventilation from tissue respiratory acidosis, improvements in sleep quality from carotid body carbonic anhydrase inhibition, and effects of diuresis .
Biochemical Analysis
Biochemical Properties
Acetazolamide D3 interacts with various enzymes and proteins, primarily through the inhibition of carbonic anhydrase . This inhibition reduces the secretion of certain fluids, decreases renal blood flow and glomerular filtration, and can act to discharge abnormal discharge or neurons in the central nervous system .
Cellular Effects
Acetazolamide D3 has a profound impact on various types of cells and cellular processes . It influences cell function by focusing on cellular membrane ion/water channel activity . It effectively reduces intracranial pressure (ICP), irrespective of the mode of drug administration and level of anaesthesia . The effect appears to occur via a direct action on the choroid plexus and an associated decrease in cerebrospinal fluid secretion .
Molecular Mechanism
The molecular mechanism of Acetazolamide D3 primarily involves the inhibition of carbonic anhydrase . This leads to an increased transneuronal chloride gradient, increased chloride current, and increased inhibition . It also affects the pharmacokinetics of other drugs .
Temporal Effects in Laboratory Settings
In laboratory settings, the effects of Acetazolamide D3 change over time . It has been observed that the prolonged use of Acetazolamide D3 is likely to be limited due to common adverse events . A better understanding of Acetazolamide D3’s clinical impact and molecular mechanisms is sure to elaborate on both healthy physiological mechanisms and disease .
Dosage Effects in Animal Models
In animal models, the effects of Acetazolamide D3 vary with different dosages . For instance, in dogs, the usual dose is 3.5 to 5 mg per pound every 6 hours when treating metabolic acidosis or glaucoma . In cats, the usual dose is 3.5 mg per pound every 8 hours .
Metabolic Pathways
Acetazolamide D3 is involved in various metabolic pathways . It inhibits carbonic anhydrase, causing a reduction in the availability of hydrogen ions for active transport in the renal tubule lumen . This leads to alkaline urine and an increase in the excretion of bicarbonate, sodium, potassium, and water .
Transport and Distribution
Acetazolamide D3 is transported and distributed within cells and tissues . After oral ingestion, Acetazolamide D3 is rapidly absorbed with a bioavailability of >90% . Its volume of distribution is 0.3 L/kg, and plasma protein binding is 90–95% . It is not metabolized and approximately 100% of an administered dose is excreted as unchanged Acetazolamide D3 in urine .
Subcellular Localization
It is known that Acetazolamide D3 can aggregate in distinct patterns within various cellular compartments
Preparation Methods
Synthetic Routes and Reaction Conditions
The synthesis of acetazolamide-d3 involves the incorporation of deuterium atoms into the acetazolamide molecule. This can be achieved through various synthetic routes, including the use of deuterated reagents and solvents. One common method involves the reaction of deuterated acetic anhydride with 5-amino-1,3,4-thiadiazole-2-sulfonamide under controlled conditions to yield this compound .
Industrial Production Methods
Industrial production of this compound typically involves large-scale synthesis using deuterated reagents. The process includes multiple steps of purification and quality control to ensure the high purity and isotopic enrichment of the final product. Techniques such as high-performance liquid chromatography (HPLC) and mass spectrometry are used to verify the product’s quality .
Chemical Reactions Analysis
Types of Reactions
Acetazolamide-d3 undergoes various chemical reactions, including:
Oxidation: Conversion of thiol derivatives to sulfonyl chlorides.
Reduction: Reduction of nitro groups to amines.
Substitution: Nucleophilic substitution reactions involving sulfonamide groups.
Common Reagents and Conditions
Oxidation: Sodium hypochlorite or chlorine gas.
Reduction: Hydrogen gas with a palladium catalyst.
Substitution: Various nucleophiles such as amines and hydrazones.
Major Products Formed
The major products formed from these reactions include sulfonamide derivatives, which have significant biological and pharmacological activities .
Scientific Research Applications
Acetazolamide-d3 is widely used in scientific research for various applications:
Biology: Studying the metabolic pathways and pharmacokinetics of acetazolamide.
Medicine: Investigating the therapeutic effects and side effects of acetazolamide in clinical trials.
Industry: Quality control and validation of pharmaceutical formulations containing acetazolamide.
Comparison with Similar Compounds
Similar Compounds
Methazolamide: Another carbonic anhydrase inhibitor used to treat glaucoma.
Dorzolamide: A topical carbonic anhydrase inhibitor used in eye drops for glaucoma.
Brinzolamide: Similar to dorzolamide, used for reducing intraocular pressure.
Uniqueness
Acetazolamide-d3 is unique due to its deuterium labeling, which provides enhanced stability and allows for precise quantification in mass spectrometry. This makes it an invaluable tool in pharmacokinetic studies and quality control processes .
Properties
IUPAC Name |
2,2,2-trideuterio-N-(5-sulfamoyl-1,3,4-thiadiazol-2-yl)acetamide | |
---|---|---|
Source | PubChem | |
URL | https://pubchem.ncbi.nlm.nih.gov | |
Description | Data deposited in or computed by PubChem | |
InChI |
InChI=1S/C4H6N4O3S2/c1-2(9)6-3-7-8-4(12-3)13(5,10)11/h1H3,(H2,5,10,11)(H,6,7,9)/i1D3 | |
Source | PubChem | |
URL | https://pubchem.ncbi.nlm.nih.gov | |
Description | Data deposited in or computed by PubChem | |
InChI Key |
BZKPWHYZMXOIDC-FIBGUPNXSA-N | |
Source | PubChem | |
URL | https://pubchem.ncbi.nlm.nih.gov | |
Description | Data deposited in or computed by PubChem | |
Canonical SMILES |
CC(=O)NC1=NN=C(S1)S(=O)(=O)N | |
Source | PubChem | |
URL | https://pubchem.ncbi.nlm.nih.gov | |
Description | Data deposited in or computed by PubChem | |
Isomeric SMILES |
[2H]C([2H])([2H])C(=O)NC1=NN=C(S1)S(=O)(=O)N | |
Source | PubChem | |
URL | https://pubchem.ncbi.nlm.nih.gov | |
Description | Data deposited in or computed by PubChem | |
Molecular Formula |
C4H6N4O3S2 | |
Source | PubChem | |
URL | https://pubchem.ncbi.nlm.nih.gov | |
Description | Data deposited in or computed by PubChem | |
DSSTOX Substance ID |
DTXSID10675501 | |
Record name | N-(5-Sulfamoyl-1,3,4-thiadiazol-2-yl)(~2~H_3_)acetamide | |
Source | EPA DSSTox | |
URL | https://comptox.epa.gov/dashboard/DTXSID10675501 | |
Description | DSSTox provides a high quality public chemistry resource for supporting improved predictive toxicology. | |
Molecular Weight |
225.3 g/mol | |
Source | PubChem | |
URL | https://pubchem.ncbi.nlm.nih.gov | |
Description | Data deposited in or computed by PubChem | |
CAS No. |
1189904-01-5 | |
Record name | N-(5-Sulfamoyl-1,3,4-thiadiazol-2-yl)(~2~H_3_)acetamide | |
Source | EPA DSSTox | |
URL | https://comptox.epa.gov/dashboard/DTXSID10675501 | |
Description | DSSTox provides a high quality public chemistry resource for supporting improved predictive toxicology. | |
Q1: Why was Acetazolamide D3 chosen as the internal standard for this LC-MS/MS assay?
A1: The research article focuses on developing a sensitive and reliable method to quantify Acetazolamide in human plasma []. Acetazolamide D3, a deuterated form of Acetazolamide, likely shares very similar physicochemical properties with the analyte. This similarity makes it an ideal internal standard for several reasons:
Disclaimer and Information on In-Vitro Research Products
Please be aware that all articles and product information presented on BenchChem are intended solely for informational purposes. The products available for purchase on BenchChem are specifically designed for in-vitro studies, which are conducted outside of living organisms. In-vitro studies, derived from the Latin term "in glass," involve experiments performed in controlled laboratory settings using cells or tissues. It is important to note that these products are not categorized as medicines or drugs, and they have not received approval from the FDA for the prevention, treatment, or cure of any medical condition, ailment, or disease. We must emphasize that any form of bodily introduction of these products into humans or animals is strictly prohibited by law. It is essential to adhere to these guidelines to ensure compliance with legal and ethical standards in research and experimentation.