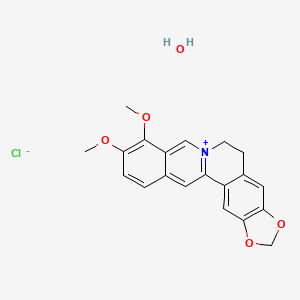
Berberine chloride hydrate
Overview
Description
Berberine chloride hydrate is a naturally occurring alkaloid derived from various plants such as Berberis, Coptis, and Hydrastis species . It has been used in traditional medicine for centuries and is gaining significant attention in the scientific and medical communities for its multifaceted therapeutic applications. This compound is known for its potential in targeting a wide array of conditions, including metabolic disorders like type 2 diabetes, cardiovascular diseases, gastrointestinal infections, and certain forms of cancer .
Mechanism of Action
Target of Action
Berberine chloride hydrate (BBR) is a naturally occurring isoquinoline alkaloid that has been identified to interact with several cellular and molecular targets . These targets include AMP-activated protein kinase (AMPK), nuclear factor κB (NF-κB), mitogen-activated protein kinase silent information regulator 1 (SIRT-1), hypoxia-inducible factor 1α (HIF-1α), vascular endothelial growth factor phosphoinositide 3-kinase (PI3K), protein kinase B (Akt), janus kinase 2 (JAK-2), Ca 2+ channels, and endoplasmic reticulum stress .
Mode of Action
BBR interacts with its targets, leading to a variety of changes in cellular function. For instance, it shows remarkable anti-inflammatory, antioxidant, antiapoptotic, and antiautophagic activity via the regulation of multiple signaling pathways . It also inhibits DNA topoisomerase .
Biochemical Pathways
BBR affects several biochemical pathways. It shows anti-inflammatory and antioxidant activity by regulating AMPK, NF-κB, and other pathways . It also impacts lipid metabolism, reducing the body’s ability to uptake long-chain fatty acids, which could prevent weight gain and cholesterol buildup . Furthermore, BBR downregulates the expression of genes involved in lipogenesis and upregulates those involved in energy expenditure in adipose tissue and muscle .
Pharmacokinetics
The pharmacokinetics of BBR is complex due to its poor bioavailability when administered orally . This is primarily due to P-glycoprotein (P-gp)-mediated efflux and self-aggregation, which greatly hinder its absorption . To overcome this, transdermal formulations of BBR have been developed, which have shown to achieve BBR levels well above oral administration .
Result of Action
The molecular and cellular effects of BBR’s action are diverse. It has been found to suppress Streptococcus mutans biofilm formation as well as its cariogenic virulence including acid production and EPS synthesis . It also reduces the antioxidant capacity of S. aureus . In human liver cells, BBR administration resulted in a dose- and time-dependent increase in InsR expression, InsR mRNA, and protein expression .
Action Environment
The action, efficacy, and stability of BBR can be influenced by environmental factors such as pH. For instance, the antibacterial effect of BBR was found to be enhanced under acidic and alkaline conditions . This suggests that the environment in which BBR is administered can significantly impact its therapeutic effectiveness.
Biochemical Analysis
Biochemical Properties
Berberine chloride hydrate plays a crucial role in various biochemical reactions. One of its primary actions is the activation of AMP-activated protein kinase (AMPK), a key enzyme in cellular energy homeostasis. By activating AMPK, this compound enhances glucose uptake in muscle cells and improves insulin sensitivity, which is particularly beneficial for individuals with type 2 diabetes . Additionally, this compound interacts with several biomolecules, including transcription factors, cytokines, and enzymes. It inhibits the activity of pro-inflammatory cytokines and reduces oxidative stress, thereby exerting anti-inflammatory effects . This compound also modulates lipid metabolism, leading to lowered cholesterol and triglyceride levels .
Cellular Effects
This compound has significant effects on various types of cells and cellular processes. It influences cell function by activating AMPK, which regulates metabolism and energy levels within cells . This activation leads to improved glucose uptake, enhanced fatty acid oxidation, and inhibited lipid synthesis . This compound also affects cell signaling pathways, gene expression, and cellular metabolism. It has been shown to reduce inflammation and oxidative stress, which can help alleviate symptoms associated with chronic inflammatory conditions such as arthritis, metabolic syndrome, and cardiovascular disease . Furthermore, this compound has antimicrobial properties, disrupting the cell wall synthesis of bacteria and making it effective against various pathogenic microorganisms .
Molecular Mechanism
The molecular mechanism of this compound involves multiple pathways. One key mechanism is the activation of AMPK, which stimulates glucose uptake, enhances fatty acid oxidation, and inhibits lipid synthesis . This compound also modulates the expression of various genes involved in tumorigenesis and inflammation, including transcription factor nuclear factor-kappa B (NF-kB), myeloid cell leukemia 1 (Mcl-1), B-cell lymphoma 2 (Bcl-2), and cyclooxygenase (COX)-2 . Additionally, it affects the activity of enzymes such as peroxisome proliferator-activated receptor (PPAR)-γ and glucose transporter (GLUT) 4, further amplifying its therapeutic potential .
Temporal Effects in Laboratory Settings
In laboratory settings, the effects of this compound can change over time. Studies have shown that this compound is stable for up to six months under various pH and temperature conditions . Its bioavailability is relatively low, and it undergoes extensive first-pass metabolism . Long-term effects on cellular function have been observed in both in vitro and in vivo studies, with this compound demonstrating sustained anti-inflammatory and antioxidant properties . Additionally, chronic administration of this compound has been shown to result in higher levels of circulating berberine and its metabolites .
Dosage Effects in Animal Models
The effects of this compound vary with different dosages in animal models. Studies have shown that this compound has a dose-dependent effect on memory improvement in animal models of Alzheimer’s disease . At higher doses, this compound has been observed to have toxic effects, including gastrointestinal discomfort and liver toxicity . At therapeutic doses, it has shown promise in treating metabolic disorders, cardiovascular diseases, and certain forms of cancer .
Metabolic Pathways
This compound is involved in several metabolic pathways. It is extensively metabolized in the liver and intestine, with phase II metabolites being the major metabolites present in blood circulation . The primary metabolic pathways include glucuronidation and sulfation, leading to the formation of metabolites such as berberrubine, demethyleneberberine, and jatrorrhizine . These metabolites contribute to the therapeutic effects of this compound by modulating metabolic flux and metabolite levels .
Transport and Distribution
This compound is transported and distributed within cells and tissues through various mechanisms. It is absorbed in the intestine and undergoes extensive first-pass metabolism in the liver . The compound is then distributed to various tissues, including the liver, kidney, and brain . This compound interacts with transporters and binding proteins, which facilitate its localization and accumulation within cells . The bioavailability of this compound is relatively low, with only a small fraction of the administered dose reaching systemic circulation .
Subcellular Localization
The subcellular localization of this compound plays a crucial role in its activity and function. Studies have shown that this compound is enriched in the mitochondria of cells . The mitochondrial membrane potential significantly enhances berberine uptake and inhibits its efflux, leading to higher intracellular concentrations . This localization is essential for its therapeutic effects, as it allows this compound to interact with mitochondrial enzymes and modulate cellular energy metabolism .
Preparation Methods
Synthetic Routes and Reaction Conditions
Berberine chloride hydrate can be synthesized through various methods. One common approach involves the extraction of berberine from plant sources followed by its conversion to the chloride salt. The extraction process typically involves the use of solvents such as ethanol or methanol to isolate berberine from plant materials. The isolated berberine is then reacted with hydrochloric acid to form berberine chloride .
Industrial Production Methods
In industrial settings, the production of this compound often involves large-scale extraction from plant sources followed by purification and crystallization processes. The extracted berberine is subjected to a series of purification steps, including filtration, evaporation, and recrystallization, to obtain high-purity this compound .
Chemical Reactions Analysis
Types of Reactions
Berberine chloride hydrate undergoes various chemical reactions, including oxidation, reduction, and substitution reactions. These reactions are often facilitated by specific reagents and conditions.
Common Reagents and Conditions
Oxidation: this compound can be oxidized using oxidizing agents such as potassium permanganate or hydrogen peroxide under acidic conditions.
Reduction: Reduction of this compound can be achieved using reducing agents like sodium borohydride or lithium aluminum hydride.
Substitution: Substitution reactions involving this compound often require nucleophilic reagents such as sodium methoxide or potassium hydroxide.
Major Products Formed
The major products formed from these reactions depend on the specific reagents and conditions used. For example, oxidation of this compound can lead to the formation of berberine oxide, while reduction can yield dihydroberberine .
Scientific Research Applications
Berberine chloride hydrate has a wide range of scientific research applications across various fields:
Chemistry
In chemistry, this compound is used as a fluorescent stain for heparin in mast cells due to its strong yellow fluorescence under ultraviolet light .
Biology
In biological research, this compound is studied for its antimicrobial properties. It has been shown to disrupt the cell wall synthesis of bacteria, making it effective against various pathogenic microorganisms .
Medicine
In medicine, this compound is being investigated for its potential therapeutic effects on metabolic disorders, cardiovascular diseases, and cancer. It has shown promise in improving insulin sensitivity, reducing cholesterol levels, and inducing apoptosis in cancer cells .
Industry
In the industrial sector, this compound is used in the production of pharmaceuticals and dietary supplements. Its ability to modulate lipid metabolism and exert anti-inflammatory effects makes it a valuable ingredient in health products .
Comparison with Similar Compounds
Berberine chloride hydrate belongs to the class of protoberberine alkaloids, which includes other compounds such as palmatine, jatrorrhizine, and coptisine . Compared to these similar compounds, this compound is unique in its ability to activate AMPK and modulate multiple biochemical pathways. This multifaceted mechanism of action makes it a versatile and potent therapeutic agent .
List of Similar Compounds
- Palmatine
- Jatrorrhizine
- Coptisine
This compound stands out due to its extensive research and potential therapeutic applications, making it a compound of significant interest in various scientific fields.
Properties
IUPAC Name |
16,17-dimethoxy-5,7-dioxa-13-azoniapentacyclo[11.8.0.02,10.04,8.015,20]henicosa-1(13),2,4(8),9,14,16,18,20-octaene;chloride;hydrate | |
---|---|---|
Source | PubChem | |
URL | https://pubchem.ncbi.nlm.nih.gov | |
Description | Data deposited in or computed by PubChem | |
InChI |
InChI=1S/C20H18NO4.ClH.H2O/c1-22-17-4-3-12-7-16-14-9-19-18(24-11-25-19)8-13(14)5-6-21(16)10-15(12)20(17)23-2;;/h3-4,7-10H,5-6,11H2,1-2H3;1H;1H2/q+1;;/p-1 | |
Source | PubChem | |
URL | https://pubchem.ncbi.nlm.nih.gov | |
Description | Data deposited in or computed by PubChem | |
InChI Key |
BPNJXFPOPCFZOC-UHFFFAOYSA-M | |
Source | PubChem | |
URL | https://pubchem.ncbi.nlm.nih.gov | |
Description | Data deposited in or computed by PubChem | |
Canonical SMILES |
COC1=C(C2=C[N+]3=C(C=C2C=C1)C4=CC5=C(C=C4CC3)OCO5)OC.O.[Cl-] | |
Source | PubChem | |
URL | https://pubchem.ncbi.nlm.nih.gov | |
Description | Data deposited in or computed by PubChem | |
Molecular Formula |
C20H20ClNO5 | |
Source | PubChem | |
URL | https://pubchem.ncbi.nlm.nih.gov | |
Description | Data deposited in or computed by PubChem | |
DSSTOX Substance ID |
DTXSID20987465 | |
Record name | 9,10-Dimethoxy-5,6-dihydro-2H-[1,3]dioxolo[4,5-g]isoquinolino[3,2-a]isoquinolin-7-ium chloride--water (1/1/1) | |
Source | EPA DSSTox | |
URL | https://comptox.epa.gov/dashboard/DTXSID20987465 | |
Description | DSSTox provides a high quality public chemistry resource for supporting improved predictive toxicology. | |
Molecular Weight |
389.8 g/mol | |
Source | PubChem | |
URL | https://pubchem.ncbi.nlm.nih.gov | |
Description | Data deposited in or computed by PubChem | |
CAS No. |
68030-18-2 | |
Record name | Benzo(g)-1,3-benzodioxolo(5,6-a)quinolizinium, 5,6-dihydro-9,10-dimethoxy-, chloride, monohydrate | |
Source | ChemIDplus | |
URL | https://pubchem.ncbi.nlm.nih.gov/substance/?source=chemidplus&sourceid=0068030182 | |
Description | ChemIDplus is a free, web search system that provides access to the structure and nomenclature authority files used for the identification of chemical substances cited in National Library of Medicine (NLM) databases, including the TOXNET system. | |
Record name | 9,10-Dimethoxy-5,6-dihydro-2H-[1,3]dioxolo[4,5-g]isoquinolino[3,2-a]isoquinolin-7-ium chloride--water (1/1/1) | |
Source | EPA DSSTox | |
URL | https://comptox.epa.gov/dashboard/DTXSID20987465 | |
Description | DSSTox provides a high quality public chemistry resource for supporting improved predictive toxicology. | |
Q1: How does Berberine chloride hydrate interact with bacterial biofilms, and what are the implications for its use in treating dental caries?
A2: BCH effectively inhibits Streptococcus mutans biofilm formation by downregulating the expression of crucial genes involved in bacterial adhesion, colonization, and biofilm formation, such as srtA, spaP, and gbpC []. This inhibitory effect is particularly relevant in the context of dental caries, where S. mutans plays a major role. By suppressing biofilm formation and reducing acid production (through downregulation of comX and ldh genes) [], BCH shows promise as a potential therapeutic agent for preventing and managing dental caries.
Q2: Can this compound be incorporated into drug delivery systems, and what are the advantages of doing so?
A3: Yes, BCH can be successfully incorporated into advanced drug delivery systems. One example is its incorporation into shape memory polyurethane (SMPU) fibers using core-sheath wet-spinning technology []. These composite fibers offer controlled and programmable release of BCH, demonstrating pH-dependent release kinetics influenced by both diffusion and drug solubility []. The ability to tailor the release rate by manipulating the fiber shape through stretching and fixation opens up exciting possibilities for personalized drug delivery applications.
Q3: What are the potential applications of this compound beyond its antibacterial effects?
A4: Research suggests that BCH holds therapeutic potential beyond its antimicrobial properties. Studies show that BCH, in combination with aerobic exercise, might offer benefits in managing diabetes-related complications []. Although specific investigations into BCH's impact on cardiac muscle mitochondrial biogenesis in diabetic rats did not reveal significant changes at the tested doses [], further research with higher doses and different training protocols is warranted. Additionally, investigations into BCH's potential against harmaline-induced motor impairments in rat models of tremor [] highlight its potential therapeutic applications in neurological disorders.
Q4: How can we visually detect Perfluorooctane sulfonate (PFOS) using this compound?
A5: Interestingly, BCH plays a role in the highly selective fluorescent visual detection of PFOS. This method utilizes blue fluorescent carbon dots and leverages the interaction between BCH and PFOS []. This highlights the versatility of BCH in analytical chemistry applications, particularly in environmental monitoring and assessment.
Q5: What is the role of this compound in psoriasis phototherapy?
A6: BCH demonstrates potential as a UV-A and UV-B blocking agent, which are crucial components in topical creams for psoriasis phototherapy []. Research indicates that a combination of BCH and quercetin hydrate exhibits suitable UV blocking properties and acceptable photostability for potential application in these formulations []. Furthermore, this combination showed comparable cytotoxicity to standard UV filters like avobenzone and octinoxate at low concentrations [], suggesting a potential for developing safer and more effective topical treatments for psoriasis.
Disclaimer and Information on In-Vitro Research Products
Please be aware that all articles and product information presented on BenchChem are intended solely for informational purposes. The products available for purchase on BenchChem are specifically designed for in-vitro studies, which are conducted outside of living organisms. In-vitro studies, derived from the Latin term "in glass," involve experiments performed in controlled laboratory settings using cells or tissues. It is important to note that these products are not categorized as medicines or drugs, and they have not received approval from the FDA for the prevention, treatment, or cure of any medical condition, ailment, or disease. We must emphasize that any form of bodily introduction of these products into humans or animals is strictly prohibited by law. It is essential to adhere to these guidelines to ensure compliance with legal and ethical standards in research and experimentation.