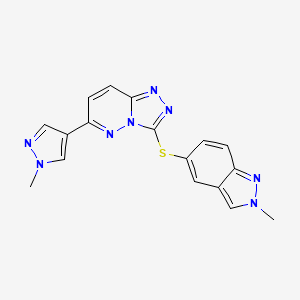
c-Met inhibitor 1
Overview
Description
c-Met inhibitor 1 is a small molecule that inhibits the enzymatic activity of the c-Met tyrosine kinase, which is the receptor for hepatocyte growth factor (HGF). This inhibition is significant because c-Met plays a crucial role in various cellular processes, including cell growth, motility, and differentiation. Dysregulation of c-Met activity is associated with the development and progression of several types of cancers, making c-Met inhibitors valuable in cancer therapy .
Mechanism of Action
- c-Met (Mesenchymal-to-Epithelial Transition Factor) : This receptor tyrosine kinase is overexpressed in various solid tumors. Its natural ligand is hepatocyte growth factor (HGF). Upon HGF binding, c-Met undergoes activation, leading to downstream signaling pathways involved in cell proliferation, angiogenesis, survival, and metastasis .
- Downstream Effects : By inhibiting c-Met, these compounds reduce tumor cell proliferation, angiogenesis, and metastasis .
- Signaling Pathways : c-Met activation triggers several signaling pathways, including Wnt/β-catenin, Ras/MAPK, PI3K/AKT, JAK/STAT, and SRC. Inhibition of c-Met disrupts these pathways, affecting cell behavior and survival .
- Impact on Bioavailability : High bioavailability ensures effective drug delivery to the target site .
Target of Action
Mode of Action
Biochemical Pathways
Pharmacokinetics
Result of Action
Biochemical Analysis
Biochemical Properties
“c-Met inhibitor 1” interacts with the c-Met receptor, which is a tyrosine kinase. It competes with ATP for binding to the kinase domain of the c-Met receptor, thereby inhibiting its phosphorylation and activation . This interaction disrupts the downstream signaling pathways of c-Met, including the PI3K/AKT, Ras/MAPK, and Wnt pathways .
Cellular Effects
“this compound” has significant effects on various types of cells. It inhibits the proliferation and migration of cancer cells . It also influences cell function by impacting cell signaling pathways, gene expression, and cellular metabolism .
Molecular Mechanism
“this compound” exerts its effects at the molecular level by binding to the c-Met receptor and inhibiting its activation . This prevents the phosphorylation of downstream proteins and disrupts several signaling pathways, including the PI3K/AKT, Ras/MAPK, and Wnt pathways .
Temporal Effects in Laboratory Settings
In laboratory settings, the effects of “this compound” change over time. It has been shown to have excellent pharmacokinetic profiles in rat in vivo studies
Metabolic Pathways
“this compound” is involved in several metabolic pathways. By inhibiting the c-Met receptor, it disrupts the PI3K/AKT, Ras/MAPK, and Wnt pathways . These pathways are crucial for cellular metabolism, and their disruption can affect metabolic flux and metabolite levels.
Transport and Distribution
It is known that c-Met inhibitors can permeate cells to reach the intracellular c-Met receptor .
Subcellular Localization
“this compound” targets the c-Met receptor, which is located on the cell surface Therefore, it is likely that “this compound” localizes to the cell membrane
Preparation Methods
Synthetic Routes and Reaction Conditions
The synthesis of c-Met inhibitor 1 typically involves the creation of a core structure, such as an indolin-2-one, followed by various substitutions to enhance its inhibitory activity. For instance, the synthesis might start with the formation of an indolinone core, which is then modified by adding different functional groups at specific positions to improve its binding affinity and selectivity for the c-Met receptor .
Industrial Production Methods
Industrial production of c-Met inhibitors involves large-scale synthesis using optimized reaction conditions to ensure high yield and purity. This often includes the use of automated synthesis equipment and stringent quality control measures to maintain consistency and efficacy of the final product .
Chemical Reactions Analysis
Types of Reactions
c-Met inhibitor 1 undergoes various chemical reactions, including:
Oxidation: This reaction can modify the functional groups on the inhibitor, potentially altering its activity.
Reduction: Reduction reactions can be used to modify the inhibitor’s structure to enhance its stability and efficacy.
Common Reagents and Conditions
Common reagents used in the synthesis of this compound include:
Oxidizing agents: Such as hydrogen peroxide or potassium permanganate.
Reducing agents: Such as sodium borohydride or lithium aluminum hydride.
Substituting agents: Such as halogens or alkyl groups.
Major Products Formed
The major products formed from these reactions are typically derivatives of the original inhibitor with enhanced properties, such as increased potency or selectivity for the c-Met receptor .
Scientific Research Applications
c-Met inhibitor 1 has a wide range of scientific research applications, including:
Chemistry: Used as a tool to study the structure and function of the c-Met receptor and its role in cellular processes.
Biology: Employed in research to understand the mechanisms of c-Met signaling and its involvement in cancer progression.
Medicine: Investigated as a potential therapeutic agent for the treatment of various cancers, including non-small cell lung cancer, gastric cancer, and renal cell carcinoma.
Industry: Utilized in the development of new cancer therapies and in the screening of potential drug candidates
Comparison with Similar Compounds
Similar Compounds
Some similar compounds to c-Met inhibitor 1 include:
Crizotinib: An ATP-competitive inhibitor of c-Met and ALK tyrosine kinases, used in the treatment of non-small cell lung cancer.
Cabozantinib: Another c-Met inhibitor that also targets VEGFR2 and is used in the treatment of medullary thyroid cancer and renal cell carcinoma.
Uniqueness
This compound is unique in its specific binding affinity and selectivity for the c-Met receptor, which may result in fewer off-target effects and improved therapeutic outcomes compared to other inhibitors. Its unique chemical structure allows for potent inhibition of c-Met activity, making it a valuable tool in cancer research and therapy .
Properties
IUPAC Name |
3-(2-methylindazol-5-yl)sulfanyl-6-(1-methylpyrazol-4-yl)-[1,2,4]triazolo[4,3-b]pyridazine | |
---|---|---|
Source | PubChem | |
URL | https://pubchem.ncbi.nlm.nih.gov | |
Description | Data deposited in or computed by PubChem | |
InChI |
InChI=1S/C17H14N8S/c1-23-10-12(8-18-23)15-5-6-16-19-20-17(25(16)22-15)26-13-3-4-14-11(7-13)9-24(2)21-14/h3-10H,1-2H3 | |
Source | PubChem | |
URL | https://pubchem.ncbi.nlm.nih.gov | |
Description | Data deposited in or computed by PubChem | |
InChI Key |
JYXHZDNTBJUJNH-UHFFFAOYSA-N | |
Source | PubChem | |
URL | https://pubchem.ncbi.nlm.nih.gov | |
Description | Data deposited in or computed by PubChem | |
Canonical SMILES |
CN1C=C(C=N1)C2=NN3C(=NN=C3SC4=CC5=CN(N=C5C=C4)C)C=C2 | |
Source | PubChem | |
URL | https://pubchem.ncbi.nlm.nih.gov | |
Description | Data deposited in or computed by PubChem | |
Molecular Formula |
C17H14N8S | |
Source | PubChem | |
URL | https://pubchem.ncbi.nlm.nih.gov | |
Description | Data deposited in or computed by PubChem | |
Molecular Weight |
362.4 g/mol | |
Source | PubChem | |
URL | https://pubchem.ncbi.nlm.nih.gov | |
Description | Data deposited in or computed by PubChem | |
Synthesis routes and methods I
Procedure details
Synthesis routes and methods II
Procedure details
Q1: What is the mechanism of action of c-Met inhibitor 1 (zgwatinib) and what are the downstream effects of its inhibition?
A1: this compound (zgwatinib) is a potent and selective inhibitor of the c-Met receptor tyrosine kinase. [, ] While the provided abstracts do not describe the detailed mechanism of zgwatinib, they indicate that it binds to c-Met and blocks its kinase activity.
Q2: What are the challenges associated with developing selective c-Met inhibitors, and how did researchers address the issue of hERG inhibition in the case of zgwatinib?
A2: A common challenge in developing kinase inhibitors is achieving selectivity for the target kinase while minimizing off-target activity, particularly against the hERG potassium channel. Inhibition of hERG can lead to cardiac toxicity, a serious safety concern. The research on zgwatinib highlights this challenge, as initial studies identified significant toxicity associated with the compound. [] To address the hERG inhibition, researchers conducted a structure-activity relationship (SAR) campaign to modify the zgwatinib structure and reduce its hERG activity while maintaining its potency against c-Met. [] This approach led to the identification of compounds with improved safety profiles.
Q3: How does the concept of structure-activity relationship (SAR) apply to the development of c-Met inhibitors like zgwatinib?
A3: SAR studies are crucial in optimizing the potency, selectivity, and pharmacological properties of drug candidates. [, ] In the context of zgwatinib, researchers explored various structural modifications to the parent molecule. By systematically altering different parts of the molecule and evaluating the impact on c-Met inhibition and hERG activity, they gained insights into the key structural features responsible for target binding and selectivity. [] This information guided further optimization, leading to the discovery of new c-Met inhibitors with improved pharmacological profiles, such as compounds 11, 12, and 39, which showed potent c-Met inhibition while minimizing hERG activity. []
Q4: The research mentions patient-derived xenograft (PDX) models for evaluating c-Met inhibitors. Why are these models considered particularly relevant for studying c-Met-targeted therapies?
A4: PDX models are highly valuable for evaluating cancer therapies as they closely mimic the complexity and heterogeneity of human tumors. [] Unlike traditional cell line-based models, PDXs retain the tumor architecture, stromal interactions, and genetic makeup of the original patient tumor. [] This is particularly important for studying c-Met inhibitors because the c-Met pathway is often influenced by the tumor microenvironment. [] The response to c-Met inhibitors can vary depending on factors like c-Met expression levels, the presence of activating mutations, and the extent of HGF production by stromal cells. [] By utilizing PDX models, researchers can gain more accurate insights into the efficacy and potential resistance mechanisms of c-Met inhibitors in a clinically relevant setting.
Disclaimer and Information on In-Vitro Research Products
Please be aware that all articles and product information presented on BenchChem are intended solely for informational purposes. The products available for purchase on BenchChem are specifically designed for in-vitro studies, which are conducted outside of living organisms. In-vitro studies, derived from the Latin term "in glass," involve experiments performed in controlled laboratory settings using cells or tissues. It is important to note that these products are not categorized as medicines or drugs, and they have not received approval from the FDA for the prevention, treatment, or cure of any medical condition, ailment, or disease. We must emphasize that any form of bodily introduction of these products into humans or animals is strictly prohibited by law. It is essential to adhere to these guidelines to ensure compliance with legal and ethical standards in research and experimentation.