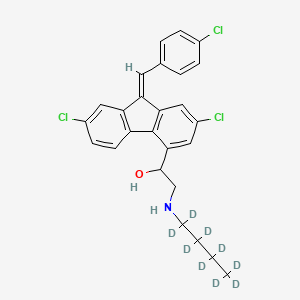
Desbutyl Lumefantrine D9
- Click on QUICK INQUIRY to receive a quote from our team of experts.
- With the quality product at a COMPETITIVE price, you can focus more on your research.
Overview
Description
Desbutyl Lumefantrine D9 is the labelled analogue of N-Desbutyl Lumefantrine, which is a metabolite of Lumefantrine .
Synthesis Analysis
A sensitive liquid chromatography tandem mass spectrometry (LC-MS/MS) method was developed for quantification of lumefantrine (LUM) and its metabolite desbutyl-lumefantrine (DBL) in human plasma .Molecular Structure Analysis
The molecular formula of Desbutyl Lumefantrine D9 is C26H24Cl3NO .Chemical Reactions Analysis
Desbutyl-lumefantrine (DBL) is a metabolite of lumefantrine. Preliminary data from Plasmodium falciparum field isolates show greater antimalarial potency than, and synergy with, the parent compound and synergy with artemisinin .Physical And Chemical Properties Analysis
Desbutyl Lumefantrine D9 has a molecular weight of 481.9 g/mol .Scientific Research Applications
Comprehensive Analysis of Desbutyl Lumefantrine D9 Applications
Desbutyl Lumefantrine D9, a metabolite of lumefantrine, plays a crucial role in the pharmacokinetics and therapeutic efficacy of antimalarial drugs. Below is a detailed analysis of its applications across various scientific research fields.
Malaria Treatment Efficacy: Desbutyl Lumefantrine D9 is a key player in assessing the efficacy of artemether–lumefantrine (AL) treatments for malaria. Studies have shown that the metabolite’s concentration in the blood correlates with the therapeutic success of AL, especially in regions with high pharmacogenetic variability .
Pharmacogenetic Research: Research on Desbutyl Lumefantrine D9 has provided insights into how genetic variations affect drug metabolism. This is particularly relevant for the CYP450 enzymes, which metabolize many antimalarial drugs. Understanding these variations can lead to more personalized and effective malaria treatments .
Pediatric Pharmacokinetics: Desbutyl Lumefantrine D9’s pharmacokinetics in children under five years of age is a critical area of study. It helps in optimizing dosing regimens for young patients, ensuring they receive the most effective concentration of the drug for treating malaria .
Antimalarial Drug Synergy: The metabolite has been observed to have greater anti-malarial potency when used in combination with lumefantrine and artemisinin. This synergy is crucial for enhancing the effectiveness of combination therapies against Plasmodium falciparum .
Drug Resistance Monitoring: Monitoring Desbutyl Lumefantrine D9 levels can serve as an indicator of emerging drug resistance. As resistance to antimalarial drugs is a growing concern, tracking the efficacy of metabolites like Desbutyl Lumefantrine D9 can help in early detection and response .
Pharmacokinetic Modeling: Developing pharmacokinetic models that include Desbutyl Lumefantrine D9 can aid in predicting drug behavior in different populations. These models are essential for understanding drug distribution, metabolism, and excretion patterns .
Clinical Trial Biomarkers: Desbutyl Lumefantrine D9 can be used as a biomarker in clinical trials to assess drug exposure and response. This application is vital for determining the safety and efficacy of new antimalarial treatments .
Global Health Initiatives: Research on Desbutyl Lumefantrine D9 contributes to global health initiatives aimed at eradicating malaria. By improving our understanding of antimalarial drug action and resistance, we can develop more effective strategies to combat this disease worldwide .
Safety and Hazards
Mechanism of Action
Target of Action
Desbutyl Lumefantrine D9 is a metabolite of Lumefantrine , an antimalarial agent primarily used in combination with artemether for the treatment of acute uncomplicated malaria caused by Plasmodium falciparum . Its primary targets are the erythrocytic stages of Plasmodium spp. .
Mode of Action
Available data suggest that lumefantrine inhibits the formation of β-hematin by forming a complex with hemin and inhibits nucleic acid and protein synthesis . As a metabolite of Lumefantrine, Desbutyl Lumefantrine D9 is expected to have similar interactions with its targets.
Biochemical Pathways
Lumefantrine and its metabolite, Desbutyl Lumefantrine D9, exert their effects by inhibiting the formation of β-hematin, a crucial step in the heme detoxification pathway of the malaria parasite . This inhibition disrupts the parasite’s ability to detoxify the heme released during hemoglobin digestion, leading to its death .
Pharmacokinetics
The pharmacokinetics of Lumefantrine and its active metabolite, Desbutyl Lumefantrine, have been characterized in children under 5 years . A two-compartment linear pharmacokinetic model incorporating lag time with first-order absorption was used to characterize the pharmacokinetics of Lumefantrine . The apparent clearance (CL/F) was explained by Body Mass Index (BMI) and age, while weight partially explained variability in the apparent volume of distribution of the central compartment (V2/F) . The absolute oral bioavailability of Lumefantrine across the tested doses ranged between 4.97% and 11.98% .
Result of Action
The result of Lumefantrine and Desbutyl Lumefantrine D9’s action is the clearance of Plasmodium spp. from the blood, effectively treating the symptoms of malaria . Lumefantrine is a blood schizonticide active against erythrocytic stages of Plasmodium falciparum . It is thought that administration of Lumefantrine with artemether results in cooperative antimalarial clearing effects .
Action Environment
The action, efficacy, and stability of Lumefantrine and its metabolite, Desbutyl Lumefantrine D9, can be influenced by various environmental factors. For instance, the clearance of Lumefantrine was found to be higher in children ≤2 years compared to those >2 years, notably decreased with increasing BMI and age from two to just less than five years . This suggests that age and BMI, which can be considered as environmental factors, significantly influence the action of Lumefantrine and its metabolite, Desbutyl Lumefantrine D9 .
properties
IUPAC Name |
1-[(9Z)-2,7-dichloro-9-[(4-chlorophenyl)methylidene]fluoren-4-yl]-2-(1,1,2,2,3,3,4,4,4-nonadeuteriobutylamino)ethanol |
Source
|
---|---|---|
Source | PubChem | |
URL | https://pubchem.ncbi.nlm.nih.gov | |
Description | Data deposited in or computed by PubChem | |
InChI |
InChI=1S/C26H24Cl3NO/c1-2-3-10-30-15-25(31)24-14-19(29)13-23-21(11-16-4-6-17(27)7-5-16)22-12-18(28)8-9-20(22)26(23)24/h4-9,11-14,25,30-31H,2-3,10,15H2,1H3/b21-11-/i1D3,2D2,3D2,10D2 |
Source
|
Source | PubChem | |
URL | https://pubchem.ncbi.nlm.nih.gov | |
Description | Data deposited in or computed by PubChem | |
InChI Key |
YLBUTQNEBVPTES-HVYWULERSA-N |
Source
|
Source | PubChem | |
URL | https://pubchem.ncbi.nlm.nih.gov | |
Description | Data deposited in or computed by PubChem | |
Canonical SMILES |
CCCCNCC(C1=CC(=CC2=C1C3=C(C2=CC4=CC=C(C=C4)Cl)C=C(C=C3)Cl)Cl)O |
Source
|
Source | PubChem | |
URL | https://pubchem.ncbi.nlm.nih.gov | |
Description | Data deposited in or computed by PubChem | |
Isomeric SMILES |
[2H]C([2H])([2H])C([2H])([2H])C([2H])([2H])C([2H])([2H])NCC(C1=CC(=CC\2=C1C3=C(/C2=C/C4=CC=C(C=C4)Cl)C=C(C=C3)Cl)Cl)O |
Source
|
Source | PubChem | |
URL | https://pubchem.ncbi.nlm.nih.gov | |
Description | Data deposited in or computed by PubChem | |
Molecular Formula |
C26H24Cl3NO |
Source
|
Source | PubChem | |
URL | https://pubchem.ncbi.nlm.nih.gov | |
Description | Data deposited in or computed by PubChem | |
Molecular Weight |
481.9 g/mol |
Source
|
Source | PubChem | |
URL | https://pubchem.ncbi.nlm.nih.gov | |
Description | Data deposited in or computed by PubChem | |
Product Name |
Desbutyl Lumefantrine D9 |
Disclaimer and Information on In-Vitro Research Products
Please be aware that all articles and product information presented on BenchChem are intended solely for informational purposes. The products available for purchase on BenchChem are specifically designed for in-vitro studies, which are conducted outside of living organisms. In-vitro studies, derived from the Latin term "in glass," involve experiments performed in controlled laboratory settings using cells or tissues. It is important to note that these products are not categorized as medicines or drugs, and they have not received approval from the FDA for the prevention, treatment, or cure of any medical condition, ailment, or disease. We must emphasize that any form of bodily introduction of these products into humans or animals is strictly prohibited by law. It is essential to adhere to these guidelines to ensure compliance with legal and ethical standards in research and experimentation.