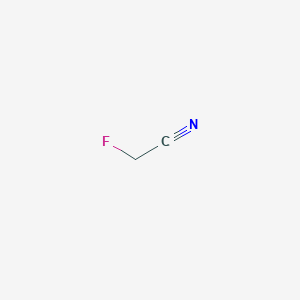
Fluoroacetonitrile
Overview
Description
Fluoroacetonitrile is an organic compound with the chemical formula C2H2FN. It is a colorless liquid with a distinctive odor. This compound is known for its applications in various fields, including organic synthesis and electrochemistry. This compound is slightly soluble in water and has a boiling point of approximately 79-80°C .
Mechanism of Action
Target of Action
Fluoroacetonitrile (FAN) is primarily used as a solvent in electric double-layer capacitors (EDLCs) . The primary targets of FAN are the components of these capacitors, where it interacts with the electrolyte to influence the overall performance of the device .
Mode of Action
FAN interacts with its targets by influencing the electrolytic conductivity and capacitance of the EDLCs . Although the electrolytic conductivity of FAN is slightly lower than that of acetonitrile (AN), another commonly used solvent, the capacitance of a model cell using FAN is higher than those obtained for AN and propylene carbonate (PC) .
Biochemical Pathways
The biochemical pathways influenced by FAN primarily involve the electrochemical processes within the EDLCs . By altering the polarity of the solvent through partial fluorination, FAN can improve the performance of these devices .
Pharmacokinetics
While the term “pharmacokinetics” is typically used in the context of drug metabolism, in the case of FAN, we can consider its properties such as relative permittivity, dynamic viscosity, and electrolytic conductivity . These properties influence its behavior in the EDLCs and impact the overall performance of the device .
Result of Action
The result of FAN’s action is an improvement in the performance of the EDLCs . Despite its slightly lower electrolytic conductivity compared to AN, the use of FAN as a solvent results in a higher capacitance for the model cell .
Action Environment
The action of FAN can be influenced by environmental factors such as temperature, which can affect its physical and electrochemical properties
Biochemical Analysis
Biochemical Properties
Fluoroacetonitrile plays a role in biochemical reactions as a reagent and intermediate. It interacts with enzymes, proteins, and other biomolecules, influencing their activity and function. For example, this compound has been shown to inhibit rat hepatic glutathione S-transferases in vitro . This interaction suggests that this compound can affect detoxification processes in the liver by modulating enzyme activity.
Cellular Effects
This compound affects various types of cells and cellular processes. It can influence cell function by altering cell signaling pathways, gene expression, and cellular metabolism. Studies have shown that this compound can cause weak inhibition of certain enzymes, which may impact metabolic pathways and cellular homeostasis . Additionally, this compound’s interaction with cellular proteins can lead to changes in cell function and viability.
Molecular Mechanism
The molecular mechanism of this compound involves its interaction with biomolecules at the molecular level. This compound can bind to enzymes and proteins, leading to inhibition or activation of their activity. For instance, this compound has been used as a reagent in the preparation of enzyme inhibitors, such as PAD4 inhibitors . These interactions can result in changes in gene expression and cellular responses, highlighting the compound’s role in modulating biochemical pathways.
Temporal Effects in Laboratory Settings
In laboratory settings, the effects of this compound can change over time. The stability and degradation of this compound are important factors that influence its long-term effects on cellular function. Studies have shown that this compound can degrade over time, leading to changes in its activity and interactions with biomolecules . Long-term exposure to this compound in in vitro or in vivo studies may result in altered cellular responses and potential toxicity.
Dosage Effects in Animal Models
The effects of this compound vary with different dosages in animal models. At low doses, this compound may have minimal impact on cellular function, while higher doses can lead to toxic or adverse effects. Studies have shown that this compound can cause dose-dependent inhibition of enzymes and changes in metabolic pathways . High doses of this compound may result in significant toxicity, affecting organ function and overall health.
Metabolic Pathways
This compound is involved in various metabolic pathways, interacting with enzymes and cofactors. It can act as a nucleophile, reacting with different substrates, including proteins and enzymes . These interactions can influence metabolic flux and metabolite levels, affecting overall cellular metabolism. The ability of this compound to modulate enzyme activity highlights its role in biochemical processes.
Transport and Distribution
Within cells and tissues, this compound is transported and distributed through various mechanisms. It can interact with transporters and binding proteins, influencing its localization and accumulation . The distribution of this compound within cells can affect its activity and function, as well as its potential toxicity. Understanding the transport and distribution of this compound is crucial for assessing its impact on cellular processes.
Subcellular Localization
This compound’s subcellular localization plays a significant role in its activity and function. It can be directed to specific compartments or organelles through targeting signals or post-translational modifications . The localization of this compound within subcellular structures can influence its interactions with biomolecules and its overall biochemical effects. Studying the subcellular localization of this compound provides insights into its role in cellular processes.
Preparation Methods
Synthetic Routes and Reaction Conditions: Fluoroacetonitrile can be synthesized through several methods. One common method involves the reaction of chloroacetamide with potassium fluoride. The reaction is typically carried out in a polar solvent, followed by distillation and purification to obtain high-purity this compound . Another method involves the reaction of acetonitrile derivatives with inorganic fluoride salts in a polar solvent .
Industrial Production Methods: Industrial production of this compound often involves the use of inexpensive and readily available raw materials. The process typically includes the substitution reaction of acetonitrile derivatives with inorganic fluoride salts, followed by multiple distillations to achieve high purity . This method is favored for its high yield, low production cost, and environmental friendliness.
Chemical Reactions Analysis
Types of Reactions: Fluoroacetonitrile undergoes various chemical reactions, including substitution, reduction, and addition reactions.
Common Reagents and Conditions:
Substitution Reactions: this compound can react with nucleophiles under mild conditions to form substituted products.
Reduction Reactions: It can be reduced to fluoroethylamine using reducing agents such as lithium aluminum hydride.
Addition Reactions: this compound can participate in addition reactions with various electrophiles to form complex organic compounds.
Major Products: The major products formed from these reactions include fluoroethylamine, fluoroacetamide, and various fluorinated organic compounds .
Scientific Research Applications
Fluoroacetonitrile has a wide range of applications in scientific research:
Comparison with Similar Compounds
Fluoroacetonitrile is unique compared to other similar compounds due to its high polarity and low viscosity. Similar compounds include:
Acetonitrile: While acetonitrile has lower polarity and viscosity, it is less effective in applications requiring high dielectric constants.
Fluoroacetamide: This compound is similar in structure but differs in its reactivity and applications.
Fluoromethyl cyanide: Another similar compound, but with different physical and chemical properties.
This compound stands out due to its unique combination of properties, making it highly valuable in various scientific and industrial applications.
Properties
IUPAC Name |
2-fluoroacetonitrile | |
---|---|---|
Source | PubChem | |
URL | https://pubchem.ncbi.nlm.nih.gov | |
Description | Data deposited in or computed by PubChem | |
InChI |
InChI=1S/C2H2FN/c3-1-2-4/h1H2 | |
Source | PubChem | |
URL | https://pubchem.ncbi.nlm.nih.gov | |
Description | Data deposited in or computed by PubChem | |
InChI Key |
GNFVFPBRMLIKIM-UHFFFAOYSA-N | |
Source | PubChem | |
URL | https://pubchem.ncbi.nlm.nih.gov | |
Description | Data deposited in or computed by PubChem | |
Canonical SMILES |
C(C#N)F | |
Source | PubChem | |
URL | https://pubchem.ncbi.nlm.nih.gov | |
Description | Data deposited in or computed by PubChem | |
Molecular Formula |
C2H2FN | |
Source | PubChem | |
URL | https://pubchem.ncbi.nlm.nih.gov | |
Description | Data deposited in or computed by PubChem | |
DSSTOX Substance ID |
DTXSID10198301 | |
Record name | Acetonitrile, fluoro- | |
Source | EPA DSSTox | |
URL | https://comptox.epa.gov/dashboard/DTXSID10198301 | |
Description | DSSTox provides a high quality public chemistry resource for supporting improved predictive toxicology. | |
Molecular Weight |
59.04 g/mol | |
Source | PubChem | |
URL | https://pubchem.ncbi.nlm.nih.gov | |
Description | Data deposited in or computed by PubChem | |
CAS No. |
503-20-8 | |
Record name | Acetonitrile, fluoro- | |
Source | CAS Common Chemistry | |
URL | https://commonchemistry.cas.org/detail?cas_rn=503-20-8 | |
Description | CAS Common Chemistry is an open community resource for accessing chemical information. Nearly 500,000 chemical substances from CAS REGISTRY cover areas of community interest, including common and frequently regulated chemicals, and those relevant to high school and undergraduate chemistry classes. This chemical information, curated by our expert scientists, is provided in alignment with our mission as a division of the American Chemical Society. | |
Explanation | The data from CAS Common Chemistry is provided under a CC-BY-NC 4.0 license, unless otherwise stated. | |
Record name | Acetonitrile, fluoro- | |
Source | ChemIDplus | |
URL | https://pubchem.ncbi.nlm.nih.gov/substance/?source=chemidplus&sourceid=0000503208 | |
Description | ChemIDplus is a free, web search system that provides access to the structure and nomenclature authority files used for the identification of chemical substances cited in National Library of Medicine (NLM) databases, including the TOXNET system. | |
Record name | Acetonitrile, fluoro- | |
Source | EPA DSSTox | |
URL | https://comptox.epa.gov/dashboard/DTXSID10198301 | |
Description | DSSTox provides a high quality public chemistry resource for supporting improved predictive toxicology. | |
Retrosynthesis Analysis
AI-Powered Synthesis Planning: Our tool employs the Template_relevance Pistachio, Template_relevance Bkms_metabolic, Template_relevance Pistachio_ringbreaker, Template_relevance Reaxys, Template_relevance Reaxys_biocatalysis model, leveraging a vast database of chemical reactions to predict feasible synthetic routes.
One-Step Synthesis Focus: Specifically designed for one-step synthesis, it provides concise and direct routes for your target compounds, streamlining the synthesis process.
Accurate Predictions: Utilizing the extensive PISTACHIO, BKMS_METABOLIC, PISTACHIO_RINGBREAKER, REAXYS, REAXYS_BIOCATALYSIS database, our tool offers high-accuracy predictions, reflecting the latest in chemical research and data.
Strategy Settings
Precursor scoring | Relevance Heuristic |
---|---|
Min. plausibility | 0.01 |
Model | Template_relevance |
Template Set | Pistachio/Bkms_metabolic/Pistachio_ringbreaker/Reaxys/Reaxys_biocatalysis |
Top-N result to add to graph | 6 |
Feasible Synthetic Routes
Q1: What is the molecular formula, weight, and spectroscopic data for Fluoroacetonitrile?
A1: this compound (C2H2FN) has a molecular weight of 59.04 g/mol. Its millimeterwave spectrum has been extensively studied []. The rotational constants, quartic and sextic centrifugal distortion constants have been determined using both the symmetric top reduction by van Eijck and Typke and the s-reduction of Watson [].
Q2: How does this compound behave as a solvent in electrochemical applications?
A2: this compound (FAN) exhibits potential as an alternative solvent for electric double-layer capacitors (EDLCs) []. While its electrolytic conductivity is slightly lower compared to acetonitrile, a model cell using FAN demonstrated higher capacitance than those using acetonitrile or propylene carbonate []. This suggests that FAN, due to its higher polarity, might enhance EDLC performance [].
Q3: Is this compound stable at high temperatures?
A3: Under neutral conditions, 2-(2-naphthyl)-2-fluoroacetonitrile, a specific α-aryl-α-fluoroacetonitrile, demonstrates configurational stability when heated up to 130°C for 6 hours [].
Q4: Can this compound be used in asymmetric synthesis?
A4: Yes, 2-aryl-2-fluoroacetonitriles are gaining interest as versatile building blocks in asymmetric synthesis []. A palladium(phosphinoxazoline) catalyzed method has been developed for the asymmetric allylic alkylation of α-aryl-α-fluoroacetonitriles []. This reaction allows for the formation of a C-C bond and the incorporation of two adjacent chiral centers, resulting in moderate to good yields, high enantioselectivities, and a diastereomeric ratio of up to 15:1 [].
Q5: How does this compound react with silicon surfaces?
A5: this compound interacts with the Si(100)-2×1 surface through a C≡N [2 + 2] cycloaddition-like reaction []. This is in contrast to chloroacetonitrile, which exhibits both C≡N [2 + 2] cycloaddition-like and ene-like reactions, and bromoacetonitrile, which exclusively undergoes the ene-like reaction []. This difference in reactivity highlights the impact of halogen substituents on the surface reaction pathways of bifunctional molecules on silicon surfaces [].
Q6: What is the electronic ground state of cyanofluorocarbene (FCCN)?
A6: Negative-ion photoelectron imaging studies have revealed that cyanofluorocarbene (FCCN) possesses a closed-shell singlet ground state []. This information, combined with experimental data, allowed for the estimation of its adiabatic electron affinity (EA = 2.081 ± 0.002 eV) and singlet-triplet gap (ΔE(S-T) = 0.42 ± 0.04 eV) [].
Q7: How do halogen substituents affect the reactivity of halogenated acetonitriles on silicon surfaces?
A7: The type of halogen substituent significantly influences the reaction pathway of halogenated acetonitriles (X−CH2C≡N, X = F, Cl, Br) on Si(100)-2×1 surfaces []. While this compound favors a C≡N [2 + 2] cycloaddition-like reaction, chloroacetonitrile exhibits both [2 + 2] cycloaddition-like and ene-like reactions, and bromoacetonitrile exclusively undergoes the ene-like reaction []. This suggests that the halogen substituent plays a key role in dictating the reaction pathway of these molecules on silicon surfaces [].
Q8: How does the structure of α-(fluoromethyl)dehydroornithine and α-(fluoromethyl)dehydroputrescine impact their inhibitory activity?
A8: (E)-Dehydro analogues of α-(fluoromethyl)putrescine and -ornithine are significantly more potent inhibitors of ornithine decarboxylase (ODC) than their saturated counterparts []. This enhanced potency is particularly pronounced in the α-fluoromethyl series []. This difference highlights the impact of structural modifications, specifically the presence of the double bond, on the inhibitory activity of these compounds [].
Q9: Can the configurational stability of α-aryl-α-fluoroacetonitriles be influenced?
A9: While 2-(2-naphthyl)-2-fluoroacetonitrile is stable at high temperatures under neutral conditions [], it undergoes complete racemization within 10 hours in the presence of stoichiometric amounts of a guanidine base at room temperature []. This suggests that the presence of specific bases can significantly affect the configurational stability of these compounds [].
Q10: What analytical methods are available for separating enantiomers of 2-aryl-2-fluoroacetonitriles?
A10: High-performance liquid chromatography (HPLC) with chiral stationary phases has proven effective for the baseline enantioseparation of various 2-aryl-2-fluoroacetonitriles []. Notably, Chiralcel OD, Chiralpak AD, and Chiralpak AS demonstrated broad applicability, while Chiralcel OJ enabled the preparative separation of 2-(2-naphthyl)-2-fluoroacetonitrile enantiomers [].
Q11: How can the structure of this compound complexes be determined?
A11: X-ray crystallography has been successfully employed to elucidate the structure of the 1,4,7,10,13,16-hexaoxacyclooctadecane (18-crown-6) bis(this compound) complex [].
Disclaimer and Information on In-Vitro Research Products
Please be aware that all articles and product information presented on BenchChem are intended solely for informational purposes. The products available for purchase on BenchChem are specifically designed for in-vitro studies, which are conducted outside of living organisms. In-vitro studies, derived from the Latin term "in glass," involve experiments performed in controlled laboratory settings using cells or tissues. It is important to note that these products are not categorized as medicines or drugs, and they have not received approval from the FDA for the prevention, treatment, or cure of any medical condition, ailment, or disease. We must emphasize that any form of bodily introduction of these products into humans or animals is strictly prohibited by law. It is essential to adhere to these guidelines to ensure compliance with legal and ethical standards in research and experimentation.