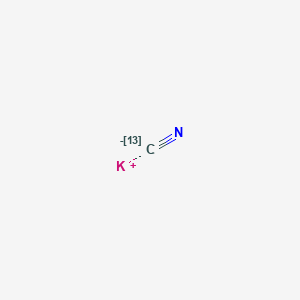
Potassium cyanide-13C
Overview
Description
Potassium cyanide-13C is a labeled compound where the carbon atom in the cyanide group is replaced with the carbon-13 isotope. This compound is used extensively in scientific research due to its unique properties, particularly in the fields of organic synthesis and isotopic labeling. This compound is a colorless crystalline solid that is highly soluble in water and has a faint, bitter almond-like odor.
Mechanism of Action
Target of Action
Potassium Cyanide-13C, like its non-isotopic counterpart, primarily targets the cytochrome c oxidase in mitochondria . This enzyme plays a crucial role in the electron transport chain, a key process in cellular respiration .
Mode of Action
This compound inhibits the cytochrome c oxidase enzyme by binding to the iron within the heme group . This binding prevents the transfer of electrons to oxygen, a process necessary for the production of ATP . As a result, the cell’s energy production is halted, leading to cell death .
Biochemical Pathways
The inhibition of cytochrome c oxidase disrupts the electron transport chain, halting the process of oxidative phosphorylation . This disruption prevents the cell from producing ATP, the primary energy currency of the cell . The lack of ATP leads to a failure of energy-dependent processes within the cell, resulting in cell death .
Pharmacokinetics
It is highly soluble in water, which suggests it can be readily absorbed and distributed in the body . It is metabolized into less toxic products, which are then excreted .
Result of Action
The primary result of this compound action is cell death due to the inhibition of ATP production . This can lead to symptoms of cyanide poisoning, including headache, confusion, nausea, and can be fatal in high doses .
Action Environment
The action of this compound can be influenced by various environmental factors. For instance, its toxicity can be increased in environments with low oxygen levels . Additionally, the presence of other substances, such as certain metal ions, can influence its stability and reactivity .
Preparation Methods
Synthetic Routes and Reaction Conditions
Potassium cyanide-13C is typically synthesized by treating hydrogen cyanide-13C with an aqueous solution of potassium hydroxide. The reaction is as follows:
HCN-13C+KOH→KCN-13C+H2O
This reaction is carried out under controlled conditions to ensure the purity and yield of the product. The solution is then evaporated under vacuum to obtain the solid this compound.
Industrial Production Methods
Industrial production of this compound follows a similar route but on a larger scale. The process involves the careful handling of hydrogen cyanide-13C and potassium hydroxide to prevent any hazardous exposure. The final product is packaged and stored under conditions that prevent moisture absorption and degradation.
Chemical Reactions Analysis
Types of Reactions
Potassium cyanide-13C undergoes various types of chemical reactions, including:
Nucleophilic Substitution: this compound acts as a nucleophile, attacking electrophilic carbon atoms in organic molecules to form nitriles.
Oxidation: It can be oxidized to form cyanate or other related compounds.
Reduction: Under certain conditions, this compound can be reduced to form simpler compounds.
Common Reagents and Conditions
Nucleophilic Substitution: Common reagents include alkyl halides, and the reaction is typically carried out in polar aprotic solvents like dimethyl sulfoxide.
Oxidation: Reagents such as hydrogen peroxide or potassium permanganate can be used under controlled conditions.
Reduction: Reducing agents like lithium aluminum hydride can be employed.
Major Products
Nitriles: Formed from nucleophilic substitution reactions.
Cyanates: Resulting from oxidation reactions.
Simpler Compounds: Produced through reduction processes.
Scientific Research Applications
Potassium cyanide-13C is widely used in scientific research due to its isotopic labeling, which allows for the tracking and analysis of chemical reactions and biological processes. Some key applications include:
Organic Synthesis: Used in the preparation of nitriles, carboxylic acids, and hydantoins.
Biological Studies: Employed in metabolic studies to trace the incorporation of carbon-13 into biological molecules.
Medical Research: Utilized in the development of diagnostic tools and therapeutic agents.
Industrial Applications: Applied in electroplating, gold mining, and the production of specialty chemicals.
Comparison with Similar Compounds
Similar Compounds
Potassium cyanide: The non-labeled version of potassium cyanide-13C.
Sodium cyanide: Another cyanide salt with similar properties but different cation.
Trimethylsilyl cyanide: A cyanide source used in organic synthesis.
Zinc cyanide: Used in electroplating and as a reagent in organic synthesis.
Uniqueness
This compound is unique due to its isotopic labeling, which allows for precise tracking and analysis in scientific research. This property makes it invaluable in studies requiring detailed understanding of reaction mechanisms and metabolic pathways.
Properties
IUPAC Name |
potassium;azanylidyne(113C)methane | |
---|---|---|
Source | PubChem | |
URL | https://pubchem.ncbi.nlm.nih.gov | |
Description | Data deposited in or computed by PubChem | |
InChI |
InChI=1S/CN.K/c1-2;/q-1;+1/i1+1; | |
Source | PubChem | |
URL | https://pubchem.ncbi.nlm.nih.gov | |
Description | Data deposited in or computed by PubChem | |
InChI Key |
NNFCIKHAZHQZJG-YTBWXGASSA-N | |
Source | PubChem | |
URL | https://pubchem.ncbi.nlm.nih.gov | |
Description | Data deposited in or computed by PubChem | |
Canonical SMILES |
[C-]#N.[K+] | |
Source | PubChem | |
URL | https://pubchem.ncbi.nlm.nih.gov | |
Description | Data deposited in or computed by PubChem | |
Isomeric SMILES |
[13C-]#N.[K+] | |
Source | PubChem | |
URL | https://pubchem.ncbi.nlm.nih.gov | |
Description | Data deposited in or computed by PubChem | |
Molecular Formula |
CKN | |
Source | PubChem | |
URL | https://pubchem.ncbi.nlm.nih.gov | |
Description | Data deposited in or computed by PubChem | |
Molecular Weight |
66.109 g/mol | |
Source | PubChem | |
URL | https://pubchem.ncbi.nlm.nih.gov | |
Description | Data deposited in or computed by PubChem | |
CAS No. |
25909-68-6 | |
Record name | 25909-68-6 | |
Source | European Chemicals Agency (ECHA) | |
URL | https://echa.europa.eu/information-on-chemicals | |
Description | The European Chemicals Agency (ECHA) is an agency of the European Union which is the driving force among regulatory authorities in implementing the EU's groundbreaking chemicals legislation for the benefit of human health and the environment as well as for innovation and competitiveness. | |
Explanation | Use of the information, documents and data from the ECHA website is subject to the terms and conditions of this Legal Notice, and subject to other binding limitations provided for under applicable law, the information, documents and data made available on the ECHA website may be reproduced, distributed and/or used, totally or in part, for non-commercial purposes provided that ECHA is acknowledged as the source: "Source: European Chemicals Agency, http://echa.europa.eu/". Such acknowledgement must be included in each copy of the material. ECHA permits and encourages organisations and individuals to create links to the ECHA website under the following cumulative conditions: Links can only be made to webpages that provide a link to the Legal Notice page. | |
Retrosynthesis Analysis
AI-Powered Synthesis Planning: Our tool employs the Template_relevance Pistachio, Template_relevance Bkms_metabolic, Template_relevance Pistachio_ringbreaker, Template_relevance Reaxys, Template_relevance Reaxys_biocatalysis model, leveraging a vast database of chemical reactions to predict feasible synthetic routes.
One-Step Synthesis Focus: Specifically designed for one-step synthesis, it provides concise and direct routes for your target compounds, streamlining the synthesis process.
Accurate Predictions: Utilizing the extensive PISTACHIO, BKMS_METABOLIC, PISTACHIO_RINGBREAKER, REAXYS, REAXYS_BIOCATALYSIS database, our tool offers high-accuracy predictions, reflecting the latest in chemical research and data.
Strategy Settings
Precursor scoring | Relevance Heuristic |
---|---|
Min. plausibility | 0.01 |
Model | Template_relevance |
Template Set | Pistachio/Bkms_metabolic/Pistachio_ringbreaker/Reaxys/Reaxys_biocatalysis |
Top-N result to add to graph | 6 |
Feasible Synthetic Routes
Disclaimer and Information on In-Vitro Research Products
Please be aware that all articles and product information presented on BenchChem are intended solely for informational purposes. The products available for purchase on BenchChem are specifically designed for in-vitro studies, which are conducted outside of living organisms. In-vitro studies, derived from the Latin term "in glass," involve experiments performed in controlled laboratory settings using cells or tissues. It is important to note that these products are not categorized as medicines or drugs, and they have not received approval from the FDA for the prevention, treatment, or cure of any medical condition, ailment, or disease. We must emphasize that any form of bodily introduction of these products into humans or animals is strictly prohibited by law. It is essential to adhere to these guidelines to ensure compliance with legal and ethical standards in research and experimentation.