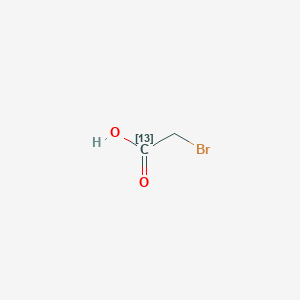
2-bromoacetic acid
Overview
Description
2-bromoacetic acid is a labeled compound where the carbon atom in the carboxyl group is replaced with the carbon-13 isotope. This compound is primarily used in research and industrial applications due to its unique isotopic labeling, which allows for detailed studies in various scientific fields .
Preparation Methods
Synthetic Routes and Reaction Conditions: 2-bromoacetic acid can be synthesized through the bromination of acetic acid-1-13C. The reaction typically involves the use of bromine in the presence of a catalyst such as phosphorus tribromide. The reaction conditions include maintaining a controlled temperature and ensuring the exclusion of moisture to prevent hydrolysis .
Industrial Production Methods: Industrial production of bromoacetic acid-1-13C follows similar synthetic routes but on a larger scale. The process involves stringent quality control measures to ensure high isotopic purity and chemical purity. The final product is often subjected to rigorous testing to confirm its isotopic enrichment and chemical composition .
Types of Reactions:
Substitution Reactions: this compound undergoes nucleophilic substitution reactions where the bromine atom is replaced by various nucleophiles such as hydroxide ions, amines, and thiols.
Oxidation and Reduction: While bromoacetic acid-1-13C is not typically involved in oxidation-reduction reactions, it can participate in reactions where the carboxyl group is reduced to an alcohol or oxidized to a carbonyl compound.
Common Reagents and Conditions:
Nucleophilic Substitution: Common reagents include sodium hydroxide, ammonia, and thiols.
Oxidation and Reduction: Reagents such as lithium aluminum hydride for reduction and potassium permanganate for oxidation are used under controlled conditions.
Major Products:
Substitution Reactions: The major products include hydroxyacetic acid, aminoacetic acid, and thioacetic acid.
Oxidation and Reduction: The major products include glycolic acid and glyoxylic acid.
Scientific Research Applications
2-bromoacetic acid has a wide range of applications in scientific research:
Mechanism of Action
The mechanism of action of bromoacetic acid-1-13C involves its ability to act as an alkylating agent. The bromine atom can be replaced by various nucleophiles, leading to the formation of new carbon-carbon or carbon-heteroatom bonds. This property makes it useful in the synthesis of complex organic molecules and in studying reaction mechanisms .
Comparison with Similar Compounds
Bromoacetic acid-2-13C: Similar to bromoacetic acid-1-13C but with the carbon-13 isotope in a different position.
Bromoacetic acid-18O2: Contains oxygen-18 isotopes instead of carbon-13.
Bromoacetic acid-13C2,d3: Contains both carbon-13 and deuterium isotopes.
Uniqueness: 2-bromoacetic acid is unique due to its specific isotopic labeling, which allows for precise tracking of the carbon atom in various chemical and biological processes. This specificity makes it particularly valuable in NMR spectroscopy and metabolic studies .
Biological Activity
2-Bromoacetic acid (BAA), also known as bromoethanoic acid, is a halogenated acetic acid derivative with significant biological activity. This compound is recognized for its various applications in biochemical research and potential therapeutic uses. This article explores the biological activity of this compound, detailing its mechanisms of action, effects on different biological systems, and relevant case studies.
- Molecular Formula : C₂H₃BrO₂
- Molecular Weight : 139.94 g/mol
- Melting Point : 47-49 °C
- Boiling Point : 208 °C
- Appearance : Crystalline solid
This compound exhibits its biological effects primarily through the following mechanisms:
- Oxidative Stress Induction : BAA has been shown to induce oxidative stress in various biological systems, leading to cellular damage. It disrupts mitochondrial function and interferes with the Nrf2 signaling pathway, which is crucial for antioxidant defense mechanisms .
- Alteration of Uric Acid Metabolism : Research indicates that BAA affects uric acid metabolism by disrupting the expression of genes involved in renal function and uric acid transport, contributing to nephrotoxicity .
- Cellular Apoptosis : BAA exposure has been associated with increased apoptosis in kidney cells, as evidenced by changes in the expression of pro-apoptotic (Bax) and anti-apoptotic (Bcl-2) proteins .
- Alkylating Agent : As a quaternizing agent, BAA can modify biomolecules, impacting protein function and potentially leading to cytotoxic effects .
Biological Effects
The biological effects of this compound have been studied in various models:
- Nephrotoxicity in Chickens : A study demonstrated that exposure to BAA resulted in kidney swelling, structural disarrangement, and functional impairment in chickens. The nephrotoxic effects were linked to oxidative stress and mitochondrial dysfunction .
- Effects on Human Peripheral Blood Mononuclear Cells (PBMCs) : BAA has been shown to induce necrotic alterations and apoptotic changes in PBMCs at millimolar concentrations, highlighting its potential immunotoxicity .
- Anticancer Properties : Some studies suggest that BAA may possess anticancer activity by inducing apoptosis in cancer cells, although the exact mechanisms remain under investigation .
Case Study 1: Nephrotoxicity in Animal Models
A study involving chickens exposed to varying concentrations of BAA (0, 3, 300, 3000 μg/L) over four weeks revealed significant renal damage. Key findings included:
- Increased serum creatinine and blood urea nitrogen levels.
- Structural changes in kidney tissues.
- Upregulation of apoptotic markers (caspase-3 activation) alongside downregulation of mitochondrial biogenesis genes .
Case Study 2: Immunotoxicity Assessment
Research assessing the impact of halogenated acetic acids (including BAA) on PBMCs found that:
- BAA induced significant necrosis and apoptosis.
- Alterations in cell morphology were noted, with increased granulation and decreased cell size observed under microscopy .
Comparative Biological Activity
The following table summarizes the biological activities of various halogenated acetic acids compared to this compound:
Compound | Anticancer Activity | Nephrotoxicity | Immunotoxicity | Mechanism |
---|---|---|---|---|
This compound | Moderate | High | High | Oxidative stress |
Dichloroacetic Acid | High | Moderate | Moderate | Pyruvate dehydrogenase activation |
Monobromoacetic Acid | Low | Low | Moderate | Alkylation |
Chloroacetic Acid | High | High | Low | Cytotoxicity |
Properties
IUPAC Name |
2-bromoacetic acid | |
---|---|---|
Source | PubChem | |
URL | https://pubchem.ncbi.nlm.nih.gov | |
Description | Data deposited in or computed by PubChem | |
InChI |
InChI=1S/C2H3BrO2/c3-1-2(4)5/h1H2,(H,4,5)/i2+1 | |
Source | PubChem | |
URL | https://pubchem.ncbi.nlm.nih.gov | |
Description | Data deposited in or computed by PubChem | |
InChI Key |
KDPAWGWELVVRCH-VQEHIDDOSA-N | |
Source | PubChem | |
URL | https://pubchem.ncbi.nlm.nih.gov | |
Description | Data deposited in or computed by PubChem | |
Canonical SMILES |
C(C(=O)O)Br | |
Source | PubChem | |
URL | https://pubchem.ncbi.nlm.nih.gov | |
Description | Data deposited in or computed by PubChem | |
Isomeric SMILES |
C([13C](=O)O)Br | |
Source | PubChem | |
URL | https://pubchem.ncbi.nlm.nih.gov | |
Description | Data deposited in or computed by PubChem | |
Molecular Formula |
C2H3BrO2 | |
Source | PubChem | |
URL | https://pubchem.ncbi.nlm.nih.gov | |
Description | Data deposited in or computed by PubChem | |
DSSTOX Substance ID |
DTXSID00443312 | |
Record name | Bromoacetic acid-1-13C | |
Source | EPA DSSTox | |
URL | https://comptox.epa.gov/dashboard/DTXSID00443312 | |
Description | DSSTox provides a high quality public chemistry resource for supporting improved predictive toxicology. | |
Molecular Weight |
139.94 g/mol | |
Source | PubChem | |
URL | https://pubchem.ncbi.nlm.nih.gov | |
Description | Data deposited in or computed by PubChem | |
CAS No. |
57858-24-9 | |
Record name | Bromoacetic acid-1-13C | |
Source | EPA DSSTox | |
URL | https://comptox.epa.gov/dashboard/DTXSID00443312 | |
Description | DSSTox provides a high quality public chemistry resource for supporting improved predictive toxicology. | |
Record name | 2-bromoacetic-(1-13C) acid | |
Source | European Chemicals Agency (ECHA) | |
URL | https://echa.europa.eu/information-on-chemicals | |
Description | The European Chemicals Agency (ECHA) is an agency of the European Union which is the driving force among regulatory authorities in implementing the EU's groundbreaking chemicals legislation for the benefit of human health and the environment as well as for innovation and competitiveness. | |
Explanation | Use of the information, documents and data from the ECHA website is subject to the terms and conditions of this Legal Notice, and subject to other binding limitations provided for under applicable law, the information, documents and data made available on the ECHA website may be reproduced, distributed and/or used, totally or in part, for non-commercial purposes provided that ECHA is acknowledged as the source: "Source: European Chemicals Agency, http://echa.europa.eu/". Such acknowledgement must be included in each copy of the material. ECHA permits and encourages organisations and individuals to create links to the ECHA website under the following cumulative conditions: Links can only be made to webpages that provide a link to the Legal Notice page. | |
Synthesis routes and methods
Procedure details
Retrosynthesis Analysis
AI-Powered Synthesis Planning: Our tool employs the Template_relevance Pistachio, Template_relevance Bkms_metabolic, Template_relevance Pistachio_ringbreaker, Template_relevance Reaxys, Template_relevance Reaxys_biocatalysis model, leveraging a vast database of chemical reactions to predict feasible synthetic routes.
One-Step Synthesis Focus: Specifically designed for one-step synthesis, it provides concise and direct routes for your target compounds, streamlining the synthesis process.
Accurate Predictions: Utilizing the extensive PISTACHIO, BKMS_METABOLIC, PISTACHIO_RINGBREAKER, REAXYS, REAXYS_BIOCATALYSIS database, our tool offers high-accuracy predictions, reflecting the latest in chemical research and data.
Strategy Settings
Precursor scoring | Relevance Heuristic |
---|---|
Min. plausibility | 0.01 |
Model | Template_relevance |
Template Set | Pistachio/Bkms_metabolic/Pistachio_ringbreaker/Reaxys/Reaxys_biocatalysis |
Top-N result to add to graph | 6 |
Feasible Synthetic Routes
Q1: What is the significance of using Bromoacetic acid-1-¹³C in the synthesis of ¹³C-labelled 2,4-diamino-6-methylpteridine?
A1: Bromoacetic acid-1-¹³C serves as a specific precursor for introducing a ¹³C atom at defined positions within the 2,4-diamino-6-methylpteridine molecule []. This specific labelling is crucial for studying the molecule's behavior and interactions using techniques like ¹³C-NMR, providing insights into its biological activity and metabolic pathways.
Q2: How is the incorporation of Bromoacetic acid-1-¹³C confirmed in the final product?
A2: The presence and location of the ¹³C atom, originating from Bromoacetic acid-1-¹³C, are confirmed through ¹³C-NMR and ¹H-NMR analyses of the synthesized 2,4-diamino-6-methylpteridine []. These techniques allow researchers to precisely identify the specific carbon atom that is ¹³C-labelled, verifying the successful incorporation of Bromoacetic acid-1-¹³C into the desired position within the molecule.
Disclaimer and Information on In-Vitro Research Products
Please be aware that all articles and product information presented on BenchChem are intended solely for informational purposes. The products available for purchase on BenchChem are specifically designed for in-vitro studies, which are conducted outside of living organisms. In-vitro studies, derived from the Latin term "in glass," involve experiments performed in controlled laboratory settings using cells or tissues. It is important to note that these products are not categorized as medicines or drugs, and they have not received approval from the FDA for the prevention, treatment, or cure of any medical condition, ailment, or disease. We must emphasize that any form of bodily introduction of these products into humans or animals is strictly prohibited by law. It is essential to adhere to these guidelines to ensure compliance with legal and ethical standards in research and experimentation.