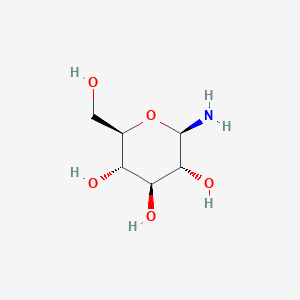
beta-D-Glucopyranosylamine
Overview
Description
β-D-Glucopyranosylamine (CAS: 7284-37-9; molecular formula: C₆H₁₃NO₅) is a glucose derivative in which the hydroxyl group at the anomeric carbon (C1) is replaced by an amine group. This structural modification confers unique biochemical properties, particularly as a competitive inhibitor of glycogen phosphorylase (GP), an enzyme critical in glycogenolysis. Its inhibitory activity is attributed to binding at the catalytic site of GP, stabilizing the inactive T-state conformation, thereby reducing glucose release .
Preparation Methods
Synthetic Routes and Reaction Conditions:
Condensation Method: One common method for synthesizing beta-D-Glucopyranosylamine involves the condensation of glucose with ammonia or an amine under acidic conditions. This reaction typically requires a catalyst and is carried out at elevated temperatures to facilitate the formation of the glycosidic bond.
Reductive Amination: Another method involves the reductive amination of glucose using an amine and a reducing agent such as sodium cyanoborohydride. This method is advantageous due to its mild reaction conditions and high yield.
Industrial Production Methods: Industrial production of this compound often involves the use of enzymatic processes to ensure high specificity and yield. Enzymes such as glycosyltransferases can be employed to catalyze the formation of the glycosidic bond under controlled conditions, making the process more efficient and environmentally friendly.
Chemical Reactions Analysis
Types of Reactions:
Oxidation: Beta-D-Glucopyranosylamine can undergo oxidation reactions to form various oxidized derivatives. Common oxidizing agents include hydrogen peroxide and potassium permanganate.
Reduction: The compound can be reduced to form corresponding amines or alcohols using reducing agents such as lithium aluminum hydride.
Substitution: this compound can participate in substitution reactions where the amino group is replaced by other functional groups. This can be achieved using reagents like acyl chlorides or sulfonyl chlorides.
Common Reagents and Conditions:
Oxidation: Hydrogen peroxide, potassium permanganate, and other oxidizing agents.
Reduction: Lithium aluminum hydride, sodium borohydride.
Substitution: Acyl chlorides, sulfonyl chlorides, and other electrophilic reagents.
Major Products:
Oxidation: Oxidized derivatives such as glucosylamines.
Reduction: Reduced amines or alcohols.
Substitution: Various substituted glucosylamines depending on the reagent used.
Scientific Research Applications
Glycogen Phosphorylase Inhibition
Mechanism of Action
Beta-D-Glucopyranosylamine and its derivatives have been extensively studied for their ability to inhibit glycogen phosphorylase, which plays a pivotal role in glycogenolysis, the process of breaking down glycogen into glucose. Dysregulation of GP is linked to conditions such as type 2 diabetes and certain cancers, making it a critical target for therapeutic intervention.
Case Studies
- A study demonstrated that N-(β-D-glucopyranosyl)-3-aryl-1,2,4-triazole-5-carboxamides exhibited low micromolar inhibition of GP, suggesting potential for developing new treatments for type 2 diabetes mellitus (T2DM) . The compounds were synthesized via amide bond formation with per-O-acetylated this compound.
- Another investigation focused on the synthesis of 2-substituted-5-(β-D-glucopyranosyl)pyrimidin-4-ones, which were found to be potent inhibitors of rabbit muscle GP with a Ki value as low as 71 nM . This highlights the effectiveness of this compound derivatives in modulating GP activity.
Structural Insights and Drug Design
Structure-Based Drug Design
The structural characteristics of this compound derivatives have been leveraged in drug design. For instance, N-acetyl-beta-D-glucopyranosylamine has been identified as a potent inhibitor that binds competitively at the catalytic site of GP . Crystallographic studies revealed that it stabilizes the T-state conformation of GP, providing insights into its binding dynamics.
Computational Studies
In silico studies using molecular docking techniques have predicted strong binding affinities for various this compound analogs, reinforcing their potential as effective GP inhibitors . These computational analyses facilitate the rational design of new compounds with enhanced inhibitory properties.
Potential Applications in Cancer Therapy
Targeting Cancer Metabolism
The expression of glycogen phosphorylase has been observed in several cancer types, including glioblastoma. Inhibiting GP could potentially disrupt the metabolic pathways that support tumor growth . Research is ongoing to explore how this compound derivatives can be utilized in cancer treatment strategies.
Summary of Research Findings
The following table summarizes key findings related to the applications of this compound:
Mechanism of Action
Beta-D-Glucopyranosylamine exerts its effects primarily through its interaction with enzymes and proteins. For instance, as an inhibitor of glycogen phosphorylase, it binds to the active site of the enzyme, preventing the breakdown of glycogen into glucose . This inhibition helps regulate blood glucose levels, making it beneficial for managing diabetes. The compound’s ability to form stable glycosidic bonds also allows it to interact with other biomolecules, influencing various biochemical pathways.
Comparison with Similar Compounds
Comparison with Structurally Related Compounds
The inhibitory efficacy and structural features of β-D-glucopyranosylamine derivatives vary significantly depending on substituents at the N-glycosylamine and hydroxyl positions. Below is a detailed analysis:
Table 1: Comparative Overview of β-D-Glucopyranosylamine Derivatives
Key Findings:
N-Acyl Derivatives :
- N-Acetyl substitution significantly enhances inhibitory potency (Ki = 32 μM) by forming hydrophobic interactions and hydrogen bonds with GP residues (e.g., Asn284, Asp338) .
- N-Trifluoroacetyl substitution reduces potency (Ki = 75 μM) due to steric hindrance and electronic effects, highlighting the sensitivity of the GP active site to substituent size .
4,6-O-Substituted Derivatives :
- The 4,6-O-butylidene group restricts ring flexibility, stabilizing the 4C₁ chair conformation and improving binding via intramolecular hydrogen bonds (e.g., O-H⋯N interactions) .
Oxalyl Derivatives :
- Despite introducing additional hydrogen-bonding capacity, oxalyl derivatives exhibit weaker inhibition (Ki = 0.2–1.4 mM), suggesting suboptimal alignment with the GP active site .
Stereochemical Influence: The β-anomeric configuration of glucopyranosylamine derivatives is critical for binding, as α-D-glucose (Ki = 1.7 mM) shows negligible inhibition .
Structural Determinants of Activity
- Hydrogen Bonding : The C1-NH group and hydroxyls at C2, C3, and C4 form hydrogen bonds with GP, stabilizing the T-state .
- Conformational Rigidity : Substituents like 4,6-O-butylidene enforce a 4C₁ chair conformation, optimizing binding .
- Hydrophobic Interactions : Acyl groups (e.g., acetyl) enhance affinity by interacting with hydrophobic pockets in the GP active site .
Biological Activity
Beta-D-Glucopyranosylamine (β-D-GPA) is a compound that has garnered attention in various fields, particularly in biochemistry and pharmacology, due to its significant biological activities. This article provides a comprehensive overview of its biological activity, mechanisms of action, and potential applications based on diverse research findings.
This compound is an amine derivative of β-D-glucopyranose, characterized by the presence of an amino group attached to the glucose molecule. Its chemical structure allows it to participate in various biochemical reactions, making it a valuable compound in both synthetic chemistry and biological research.
Target Enzyme
The primary target of β-D-GPA is glycogen phosphorylase (GP) , specifically the muscle isoform. This enzyme plays a crucial role in glycogenolysis, the biochemical pathway responsible for breaking down glycogen into glucose. Inhibition of GP can lead to decreased glucose levels in the bloodstream, which is particularly relevant for conditions such as type 2 diabetes mellitus (T2DM) .
Mode of Action
β-D-GPA acts as an inhibitor of GP, with studies indicating that it can bind to the enzyme and prevent its activity. This inhibition can help regulate blood sugar levels by reducing glycogen breakdown . The compound's interaction with GP is facilitated through its structural properties, which allow for stable binding at the enzyme's active site.
Antidiabetic Potential
Research has highlighted β-D-GPA's potential as a therapeutic agent for T2DM. By inhibiting GP, it may help manage hyperglycemia effectively. Studies have shown that derivatives of β-D-GPA exhibit low micromolar inhibition constants against GP, indicating strong inhibitory potential .
Anti-inflammatory Effects
In addition to its role in glucose metabolism, β-D-GPA has been noted for its anti-inflammatory properties. It has been suggested that this compound can modulate inflammatory responses, making it a candidate for treating autoimmune diseases and other inflammatory conditions .
Research Findings and Case Studies
A variety of studies have explored the biological activities of β-D-GPA:
- Inhibition Studies : A study reported that N-azidoacetyl-β-D-glucopyranosylamine inhibited rabbit muscle glycogen phosphorylase with a Ki value of 48.7 μM, demonstrating its effectiveness as an inhibitor .
- Synthesis and Kinetics : Another research effort synthesized N-(β-D-glucopyranosyl)-3-aryl-1,2,4-triazole-5-carboxamides and evaluated their kinetics, revealing them as low micromolar inhibitors of GP . This indicates that structural modifications can enhance the inhibitory properties of β-D-GPA derivatives.
- Biological Activity Assessment : A comprehensive evaluation involving various derivatives showed that certain modifications could lead to increased efficacy against GP while also exploring their potential as anti-inflammatory agents .
Comparison with Related Compounds
Compound Name | Inhibitory Activity (Ki or IC50) | Notes |
---|---|---|
This compound | Varies (μM range) | Primary focus on GP inhibition |
N-Acetyl-beta-D-glucopyranosylamine | Ki = 1.7 mM | Potent T-state inhibitor |
N-(β-d-glucopyranosyl)-3-aryl-1,2,4-triazole-5-carboxamides | Low micromolar | Promising for T2DM treatment |
Q & A
Basic Research Questions
Q. What are the established synthetic routes for β-D-glucopyranosylamine, and how do reaction conditions influence yield and purity?
β-D-Glucopyranosylamine is commonly synthesized via nucleophilic substitution of glucopyranosyl derivatives with ammonia or via reductive amination of reducing sugars. For example, O-peracetylated β-D-glucopyranosylamine can react with phenylurea to form biuret derivatives under controlled conditions . Key factors include solvent polarity (e.g., acetonitrile for regioselectivity), temperature (20–40°C for stability), and protecting groups (e.g., acetyl or benzoyl groups to prevent side reactions). Yield optimization requires monitoring reaction progress via TLC or HPLC, while purity is assessed using NMR (e.g., anomeric proton signals at δ 5.0–5.5 ppm) .
Q. How can researchers validate the structural identity of β-D-glucopyranosylamine derivatives?
Structural validation involves:
- NMR Spectroscopy : Confirm stereochemistry via -NMR coupling constants (e.g., for β-configuration) and -NMR anomeric carbon shifts (δ 95–105 ppm) .
- Mass Spectrometry : Use HRMS to verify molecular ion peaks (e.g., [M+Na] for glycosylamines).
- X-ray Crystallography : Resolve ambiguous cases, particularly for novel derivatives, by analyzing bond lengths and ring puckering parameters .
Advanced Research Questions
Q. How can conformational analysis resolve discrepancies in reported β-D-glucopyranosylamine ring geometries?
Ring puckering in β-D-glucopyranosylamine derivatives can be quantified using Cremer-Pople parameters (, , ), which define deviations from planarity . For instance, (puckering amplitude) and (pseudorotation phase) help distinguish chair () vs. boat () conformers. Discrepancies in crystallographic data may arise from solvent effects or intermolecular hydrogen bonding. Researchers should compare puckering coordinates across multiple datasets and use DFT calculations to model energetically favored conformers .
Q. What strategies address contradictory reactivity outcomes in glycosylamine functionalization?
Contradictions in reactivity (e.g., unexpected regioisomers) often stem from:
- Protecting Group Interference : Bulky groups (e.g., benzyl) may sterically hinder nucleophilic attack.
- Solvent Polarity : Polar aprotic solvents (e.g., DMF) favor SN2 mechanisms, while non-polar solvents may promote side reactions. Mitigation includes:
- Systematic screening of protecting groups (e.g., acetyl vs. benzoyl).
- Kinetic studies to identify rate-determining steps.
- Computational modeling (e.g., MD simulations) to predict reactive sites .
Q. How should researchers design experiments to distinguish glycosylamine tautomers in solution?
Tautomeric equilibria (e.g., open-chain vs. cyclic forms) can be studied via:
- Dynamic NMR : Observe exchange peaks in -NMR at variable temperatures.
- Isotopic Labeling : -labeling enhances detection of imine/amine transitions.
- pH-Dependent UV/Vis Spectroscopy : Monitor absorbance shifts (e.g., 240–280 nm) to track tautomerization .
Q. Methodological Considerations
Q. What safety protocols are critical when handling β-D-glucopyranosylamine in laboratory settings?
Based on MSDS
- Personal Protective Equipment (PPE) : Gloves (nitrile), lab coat, and safety goggles.
- Ventilation : Use fume hoods to avoid inhalation (harmful by inhalation, LD > 2000 mg/kg).
- Spill Management : Neutralize with inert adsorbents (e.g., vermiculite) and dispose as hazardous waste .
Q. How can researchers ensure reproducibility in glycosylamine synthesis?
- Detailed Experimental Descriptions : Follow guidelines from the Beilstein Journal of Organic Chemistry to document reagent purity (e.g., ≥98% by HPLC), reaction times, and purification steps (e.g., column chromatography with silica gel 60) .
- Open Data Practices : Share raw NMR/MS spectra and crystallographic data in repositories (e.g., RCSB PDB) to enable validation .
Q. Data Analysis and Reporting
Q. What statistical methods are recommended for analyzing glycosylamine bioactivity datasets?
- Multivariate Analysis : PCA or PLS-DA to identify structure-activity relationships (e.g., substituent effects on enzyme inhibition).
- Meta-Analysis : Aggregate data from systematic reviews (e.g., using PRISMA guidelines) to resolve conflicting bioactivity reports .
Q. How should researchers address inconsistencies in glycosylamine spectral data?
Properties
IUPAC Name |
(2R,3R,4S,5S,6R)-2-amino-6-(hydroxymethyl)oxane-3,4,5-triol | |
---|---|---|
Source | PubChem | |
URL | https://pubchem.ncbi.nlm.nih.gov | |
Description | Data deposited in or computed by PubChem | |
InChI |
InChI=1S/C6H13NO5/c7-6-5(11)4(10)3(9)2(1-8)12-6/h2-6,8-11H,1,7H2/t2-,3-,4+,5-,6-/m1/s1 | |
Source | PubChem | |
URL | https://pubchem.ncbi.nlm.nih.gov | |
Description | Data deposited in or computed by PubChem | |
InChI Key |
WCWOEQFAYSXBRK-VFUOTHLCSA-N | |
Source | PubChem | |
URL | https://pubchem.ncbi.nlm.nih.gov | |
Description | Data deposited in or computed by PubChem | |
Canonical SMILES |
C(C1C(C(C(C(O1)N)O)O)O)O | |
Source | PubChem | |
URL | https://pubchem.ncbi.nlm.nih.gov | |
Description | Data deposited in or computed by PubChem | |
Isomeric SMILES |
C([C@@H]1[C@H]([C@@H]([C@H]([C@@H](O1)N)O)O)O)O | |
Source | PubChem | |
URL | https://pubchem.ncbi.nlm.nih.gov | |
Description | Data deposited in or computed by PubChem | |
Molecular Formula |
C6H13NO5 | |
Source | PubChem | |
URL | https://pubchem.ncbi.nlm.nih.gov | |
Description | Data deposited in or computed by PubChem | |
DSSTOX Substance ID |
DTXSID20223153 | |
Record name | Glucopyranosylamine | |
Source | EPA DSSTox | |
URL | https://comptox.epa.gov/dashboard/DTXSID20223153 | |
Description | DSSTox provides a high quality public chemistry resource for supporting improved predictive toxicology. | |
Molecular Weight |
179.17 g/mol | |
Source | PubChem | |
URL | https://pubchem.ncbi.nlm.nih.gov | |
Description | Data deposited in or computed by PubChem | |
CAS No. |
7284-37-9 | |
Record name | Glucopyranosylamine | |
Source | ChemIDplus | |
URL | https://pubchem.ncbi.nlm.nih.gov/substance/?source=chemidplus&sourceid=0007284379 | |
Description | ChemIDplus is a free, web search system that provides access to the structure and nomenclature authority files used for the identification of chemical substances cited in National Library of Medicine (NLM) databases, including the TOXNET system. | |
Record name | Glucopyranosylamine | |
Source | EPA DSSTox | |
URL | https://comptox.epa.gov/dashboard/DTXSID20223153 | |
Description | DSSTox provides a high quality public chemistry resource for supporting improved predictive toxicology. | |
Retrosynthesis Analysis
AI-Powered Synthesis Planning: Our tool employs the Template_relevance Pistachio, Template_relevance Bkms_metabolic, Template_relevance Pistachio_ringbreaker, Template_relevance Reaxys, Template_relevance Reaxys_biocatalysis model, leveraging a vast database of chemical reactions to predict feasible synthetic routes.
One-Step Synthesis Focus: Specifically designed for one-step synthesis, it provides concise and direct routes for your target compounds, streamlining the synthesis process.
Accurate Predictions: Utilizing the extensive PISTACHIO, BKMS_METABOLIC, PISTACHIO_RINGBREAKER, REAXYS, REAXYS_BIOCATALYSIS database, our tool offers high-accuracy predictions, reflecting the latest in chemical research and data.
Strategy Settings
Precursor scoring | Relevance Heuristic |
---|---|
Min. plausibility | 0.01 |
Model | Template_relevance |
Template Set | Pistachio/Bkms_metabolic/Pistachio_ringbreaker/Reaxys/Reaxys_biocatalysis |
Top-N result to add to graph | 6 |
Feasible Synthetic Routes
Disclaimer and Information on In-Vitro Research Products
Please be aware that all articles and product information presented on BenchChem are intended solely for informational purposes. The products available for purchase on BenchChem are specifically designed for in-vitro studies, which are conducted outside of living organisms. In-vitro studies, derived from the Latin term "in glass," involve experiments performed in controlled laboratory settings using cells or tissues. It is important to note that these products are not categorized as medicines or drugs, and they have not received approval from the FDA for the prevention, treatment, or cure of any medical condition, ailment, or disease. We must emphasize that any form of bodily introduction of these products into humans or animals is strictly prohibited by law. It is essential to adhere to these guidelines to ensure compliance with legal and ethical standards in research and experimentation.