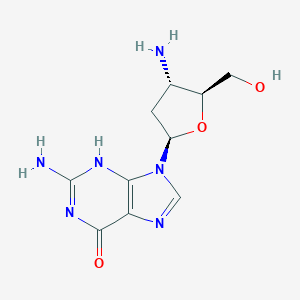
3'-Amino-2',3'-dideoxyguanosine
Overview
Description
3’-Amino-2’,3’-dideoxyguanosine is a synthetic nucleoside analog characterized by the absence of hydroxyl groups at the 2’ and 3’ positions of the ribose sugar and the presence of an amino group at the 3’ position (Figure 1). This structural modification renders it resistant to hydrolysis by cellular nucleases, making it valuable for oligonucleotide synthesis and non-enzymatic nucleic acid polymerization studies . The compound is commercially available from suppliers such as ThermoFisher, Carbosynth, and Metkinen Chemistry, and it is frequently employed in the preparation of phosphoramidites for DNA/RNA analogs with N3’→P5’ phosphoramidate linkages . Its synthesis typically involves selective protection of the 3’-amino group using reagents like Fmoc N-hydroxysuccinimide ester, achieving yields of ~81% .
Preparation Methods
Enzymatic Synthesis of 3'-Amino-2',3'-Dideoxyguanosine
Transglycosylation with Nucleoside Phosphorylases
The enzymatic synthesis of ADG begins with a transglycosylation reaction catalyzed by nucleoside phosphorylases. Bacillus stearothermophilus-derived purine nucleoside phosphorylase (PNP) and pyrimidine nucleoside phosphorylase (PyNP) are employed to transfer the 3-amino-2,3-dideoxyribose moiety from 3'-amino-3'-deoxythymidine (AMT) to 2,6-diaminopurine (DAP). This two-step process involves:
-
Phosphorolysis of AMT : PyNP cleaves the glycosidic bond of AMT, generating 3-amino-2,3-dideoxyribose-1-phosphate and thymine .
-
Ribose Transfer to DAP : PNP mediates the transfer of the phosphorylated sugar to DAP, forming 3'-amino-2',3'-dideoxy-2,6-diaminopurineriboside (DAPR) .
Optimal conditions for this stage include a reaction pH of 6.75–7.0, a temperature of 45–50°C, and a molar ratio of AMT:DAP of 1:1.1 to minimize substrate excess . Under these parameters, conversion rates exceed 90%, significantly outperforming earlier methods that relied on Escherichia coli-derived enzymes and achieved only 20.5% yields .
Deamination via Adenosine Deaminase
The intermediate DAPR undergoes deamination using Lactococcus lactis-derived adenosine deaminase (ADA), which selectively hydrolyzes the C6-amino group of DAPR to yield ADG. This reaction is conducted at a mildly alkaline pH (7.0–7.5) and 40°C for 24–120 hours, achieving near-quantitative conversion . Direct addition of ADA to the transglycosylation reaction mixture eliminates intermediate purification steps, streamlining the process and reducing production costs .
Purification and Industrial Scalability
Crystallization and Filtration
Post-reaction purification leverages the differential solubility of ADG and byproducts. Adding ethanol and a strong base (e.g., NaOH) precipitates proteins, unreacted substrates, and byproducts like thymine and guanine. The resulting mixture is filtered through a 0.2 µm membrane to remove particulate matter, yielding a crude ADG crystallite with ~85% purity .
Charcoal Treatment and Recrystallization
Further purification involves treating the crystallite with basic activated charcoal (0.05–0.3 g per gram of AMT) at 70–80°C. This step adsorbs residual impurities, increasing purity to >98%. Recrystallization from hot water-ethanol mixtures produces pharmaceutical-grade ADG with minimal loss of product .
Comparative Analysis of Traditional vs. Modern Methods
Parameter | Traditional Method (Zaitseva et al.) | Modern Enzymatic Method |
---|---|---|
Enzymes Used | E. coli BMT-38 crude extract | B. stearothermophilus PNP/PyNP, L. lactis ADA |
Reaction Yield | 20.5% | 80–90% |
Purification | Ion exchange resin | Ethanol/NaOH precipitation |
Process Time | 28 hours (reaction) + 48 hours (purification) | 24–120 hours (single batch) |
Scalability | Limited by resin capacity | Suitable for multi-kilogram batches |
Table 1: Key differences between traditional and modern ADG synthesis methods .
Enzyme Optimization and Reaction Engineering
Enzyme Sources and Thermostability
Bacillus stearothermophilus PNP and PyNP exhibit exceptional thermostability, retaining >80% activity after 24 hours at 50°C. This stability allows prolonged reaction durations without enzyme replenishment, critical for continuous production systems . In contrast, E. coli-derived enzymes degrade rapidly above 40°C, necessitating frequent batch restarts .
pH and Temperature Profiling
The transglycosylation reaction is highly pH-sensitive, with optimal activity observed at pH 6.75–7.0. Deviations beyond this range reduce conversion rates by 30–50% due to enzyme denaturation and substrate protonation . Temperature optimization studies reveal that 45°C balances reaction speed and enzyme longevity, achieving a 92% conversion rate within 12 hours .
Industrial Applications and Cost Efficiency
The modern enzymatic method reduces raw material costs by 40% compared to traditional approaches, primarily through eliminating ion exchange resins and minimizing DAP excess . A typical 100 kg batch produces 78 kg of ADG (78% yield), with production costs estimated at $12,000/kg—a 60% reduction from earlier methods .
Chemical Reactions Analysis
Types of Reactions: 3’-Amino-2’,3’-dideoxyguanosine undergoes various chemical reactions, including:
Oxidation: The amino group can be oxidized under specific conditions.
Substitution: The compound can participate in nucleophilic substitution reactions, particularly at the amino group.
Common Reagents and Conditions:
Oxidation Reagents: Common oxidizing agents include hydrogen peroxide and potassium permanganate.
Substitution Reagents: Nucleophiles such as amines and thiols are often used in substitution reactions.
Major Products: The major products formed from these reactions depend on the specific reagents and conditions used. For example, oxidation may yield corresponding oxo derivatives, while substitution reactions can produce various substituted nucleosides .
Scientific Research Applications
Target of Action
As a nucleoside analogue, 3'-Amino-ddG is incorporated into growing nucleic acid chains during replication or transcription, leading to premature termination.
Biochemical Pathways
Nucleoside analogues like 3'-Amino-ddG disrupt normal nucleic acid synthesis pathways, affecting rapidly dividing cells such as cancer cells or virus-infected cells.
Result of Action
The incorporation of 3'-Amino-ddG into nucleic acids results in cell death or inhibition of viral replication due to disruption of nucleic acid synthesis.
Scientific Research Applications
3'-Amino-ddG has a broad range of applications across various scientific disciplines:
1. Chemistry
- Used as a probe to study nucleic acid structure and function.
2. Biology
- Investigated for its role in RNA and DNA synthesis and degradation processes.
3. Medicine
- Potential therapeutic applications in antiviral research due to its ability to interfere with viral replication.
4. Industry
- Employed in the development of diagnostic tools and as a reagent in biochemical assays.
The biological activity of 3'-Amino-ddG primarily revolves around its role as an antiviral agent. Key mechanisms include:
- Chain Termination : The absence of a hydroxyl group at the 3' position prevents further elongation once incorporated into viral genomes.
- Inhibition of Viral Enzymes : It inhibits viral polymerases crucial for replication.
HIV Research
A study demonstrated that 3'-Amino-ddG effectively inhibits HIV replication in vitro. This suggests its promise as a component in combination therapies aimed at enhancing existing antiretroviral regimens.
HCV Treatment
In another investigation, 3'-Amino-ddG was evaluated against Hepatitis C Virus (HCV). Results indicated significant reductions in viral load in cell cultures, suggesting its potential as a therapeutic agent.
Research Findings
Recent studies have highlighted several important findings regarding the biological activity of 3'-Amino-ddG:
- Pharmacokinetics : The amino group enhances lipophilicity and solubility, improving absorption and bioavailability compared to other nucleoside analogs.
- Resistance Profiles : Investigations into resistance mechanisms have shown that while some viruses develop mutations against standard NRTIs, they exhibit reduced resistance to 3'-Amino-ddG, making it a valuable candidate for further development.
Mechanism of Action
The mechanism of action of 3’-Amino-2’,3’-dideoxyguanosine involves its incorporation into nucleic acids, where it can disrupt normal nucleic acid synthesis. This disruption occurs because the absence of hydroxyl groups at the 2’ and 3’ positions prevents the formation of phosphodiester bonds, thereby terminating chain elongation. The compound targets viral polymerases and other enzymes involved in nucleic acid metabolism, making it a potent inhibitor of viral replication .
Comparison with Similar Compounds
Structural and Functional Analogues
The antiviral and biochemical properties of 3’-amino-2’,3’-dideoxyguanosine are best contextualized by comparing it to structurally related dideoxyguanosine derivatives (Table 1).
Table 1. Comparative Analysis of 3’-Amino-2’,3’-Dideoxyguanosine and Analogues
Antiviral Activity and Mechanisms
- 3’-Fluoro-2’,3’-dideoxyguanosine: Exhibits potent anti-HIV activity (EC50 = 0.3–4.5 µM) by inhibiting reverse transcriptase (RT). However, it is prone to deamination by adenosine deaminase, reducing its intracellular efficacy .
- 3’-Azido-2’,3’-dideoxyguanosine (AzddG): Demonstrates sub-micromolar anti-HIV activity and a high selectivity index (>100). Resistance to AzddG in HIV-1 requires mutations like L74V, which reduce nucleotide excision by RT.
- D4G : Despite structural similarity to stavudine (D4T), D4G is inactive in cell culture, underscoring the critical role of 3’ modifications in antiviral efficacy .
Metabolism and Phosphorylation
- 3’-Amino-2’,3’-dideoxyguanosine: Primarily used as a building block in oligonucleotide synthesis rather than as a therapeutic agent. Its 3’-amino group facilitates phosphoramidate bond formation in non-enzymatic nucleic acid polymerization .
- Dideoxyguanosine analogs: Phosphorylation by cytosolic 5’-nucleotidase is critical for antiviral activity. For example, 2’,3’-dideoxyguanosine is phosphorylated with a Km of 0.9 mM, enabling conversion to its active triphosphate form .
Resistance and Clinical Relevance
Biological Activity
3'-Amino-2',3'-dideoxyguanosine (3'-Amino-ddG) is a nucleoside analog that has garnered attention for its unique structural properties and potential therapeutic applications. This article delves into its biological activity, focusing on its mechanisms, applications in antiviral research, and comparative studies with similar compounds.
Chemical Structure and Properties
3'-Amino-ddG is characterized by the absence of hydroxyl groups at the 2' and 3' positions of the ribose sugar, which are replaced by an amino group at the 3' position. This modification enhances its ability to act as a chain terminator during nucleic acid synthesis, making it particularly valuable in antiviral research.
The biological activity of 3'-Amino-ddG primarily revolves around its role as an antiviral agent. It interferes with viral replication by mimicking natural nucleosides, thereby disrupting the synthesis of viral RNA and DNA. The key mechanisms include:
- Chain Termination : The absence of a hydroxyl group at the 3' position prevents further elongation of the nucleic acid chain once incorporated into viral genomes.
- Inhibition of Viral Enzymes : It has been shown to inhibit viral polymerases, which are crucial for the replication of various viruses.
Antiviral Applications
The compound has been studied extensively for its potential use against several viral infections, particularly HIV. Research indicates that 3'-Amino-ddG exhibits significant antiviral activity, comparable to other nucleoside reverse transcriptase inhibitors (NRTIs).
Case Studies
- HIV Research : A study demonstrated that 3'-Amino-ddG effectively inhibits HIV replication in vitro, showing promise as a component in combination therapies aimed at enhancing the efficacy of existing antiretroviral regimens.
- HCV Treatment : In another investigation, 3'-Amino-ddG was evaluated for its activity against Hepatitis C Virus (HCV). Results indicated that it could reduce viral load significantly in cell cultures, suggesting its potential as a therapeutic agent .
Comparative Analysis with Similar Compounds
To better understand the unique properties of 3'-Amino-ddG, it is essential to compare it with related compounds:
Compound | Structure Characteristics | Biological Activity |
---|---|---|
This compound | Amino group at 3' position; no hydroxyl groups | Strong antiviral activity; chain terminator |
2',3'-Dideoxyguanosine | Lacks both 2' and 3' hydroxyl groups | Moderate antiviral activity; less effective than 3'-Amino-ddG |
3'-Amino-3'-deoxythymidine | Amino group at 3'; thymine instead of guanine | Limited antiviral activity; primarily used in DNA synthesis studies |
Research Findings
Recent studies have highlighted several important findings regarding the biological activity of 3'-Amino-ddG:
- Pharmacokinetics : The presence of the amino group enhances lipophilicity and solubility, improving absorption and bioavailability compared to other nucleoside analogs .
- Resistance Profiles : Investigations into resistance mechanisms have shown that while some viruses develop mutations against standard NRTIs, they exhibit reduced resistance to 3'-Amino-ddG, making it a valuable candidate for further development .
Q & A
Basic Research Questions
Q. How is 3’-Amino-2’,3’-dideoxyguanosine synthesized and structurally characterized?
Synthesis typically involves deoxygenation of precursor nucleosides. For example, Barton deoxygenation is used to remove hydroxyl groups from the ribose moiety, with protective groups (e.g., 4,4′-dimethoxytriphenylmethyl) applied to the 5′-hydroxy and 2-amino functions to prevent side reactions . Structural characterization employs 2D NMR spectroscopy to assign ¹H and ¹³C signals, particularly for the 2′,3′-dideoxy-β-D-glycero-pentofuranosyl moiety. This method confirms stereochemistry and distinguishes hydrolysis-stable N-glycosidic bonds compared to non-modified dideoxyguanosine .
Q. What is the antiviral profile of 3’-Amino-2’,3’-dideoxyguanosine?
The compound acts as a nucleoside reverse transcriptase inhibitor (NRTI), requiring intracellular phosphorylation to its active triphosphate form (3’-azido-ddGTP). It inhibits HIV-1 reverse transcriptase (RT) by competing with natural dGTP for incorporation into viral DNA, causing chain termination. Pre-steady-state kinetic studies show its incorporation efficiency (kpol/Kd) is lower than dGTP, primarily due to reduced binding affinity (Kd) rather than incorporation rate (kpol) . It also inhibits hepatitis B virus (HBV) replication in vitro, as demonstrated in duck models .
Q. What are the metabolic pathways and stability challenges of this compound?
3’-Amino-2’,3’-dideoxyguanosine undergoes sequential phosphorylation by cellular kinases to form the active triphosphate. However, its instability in solution (due to deamination or hydrolysis) and high polarity limit bioavailability. Enzymatic deamination at the 6-position of the guanine base further reduces efficacy, necessitating prodrug strategies to enhance stability .
Advanced Research Questions
Q. How do HIV-1 variants develop resistance to 3’-Amino-2’,3’-dideoxyguanosine?
Resistance arises through mutations in HIV-1 RT, such as L74V, F77L, and K476N. L74V reduces excision of the chain-terminating 3’-azido-ddGMP by impairing RT’s ability to bind ATP, a critical cofactor for excision. K476N partially restores excision activity on RNA/DNA templates by reducing secondary RNase H cleavage events, but not on DNA/DNA substrates. These mutations collectively decrease drug susceptibility by 3–4 fold, highlighting constraints in RT’s ability to evolve high-level resistance .
Q. How is this compound applied in nonenzymatic nucleic acid replication studies?
The 5′-phosphorimidazolide derivative of 2’-amino-2’,3’-dideoxyguanosine enables template-directed polymerization in protocell models. This monomer forms 2’→5’ phosphoramidate linkages on RNA or locked nucleic acid (LNA) templates, favoring A-type duplex geometry. Modifications like diaminopurine substitutions enhance polymerization efficiency, offering insights into prebiotic replication systems .
Q. What strategies improve the pharmacokinetic profile of 3’-Amino-2’,3’-dideoxyguanosine?
Prodrug modifications at the 6-position (e.g., cyclopropylamino groups) stabilize the nucleoside, increase lipophilicity, and reduce toxicity. For example, cyclo-D4G, a 6-modified prodrug, exhibits enhanced anti-HIV activity and solution stability compared to the parent compound. Triphosphate analogs (e.g., ddGTP) are synthesized via a one-pot enzymatic method for mechanistic studies .
Q. How do structural modifications influence antiviral activity and enzyme interactions?
Substitutions at the 2′,3′-positions and purine base alter RT recognition. Carbocyclic analogs (e.g., carbovir) retain anti-HIV activity with improved hydrolytic stability. Modifications like 8-aza-7-deaza substitution enhance N-glycosidic bond stability, while 2,6-diaminopurine derivatives improve base-pairing in nonenzymatic replication . Quantitative structure-activity relationship (QSAR) analysis guides optimization of hydroxyl group contributions .
Q. Methodological Considerations
3.1 Key experimental assays for studying resistance mechanisms:
- Pre-steady-state kinetics : Measure kpol (incorporation rate) and Kd (binding affinity) for 3’-azido-ddGTP vs. dGTP .
- Excision assays : Quantify ATP-mediated rescue of chain-terminated DNA synthesis using α-³²P-labeled primers .
- RNase H activity profiling : Compare cleavage efficiency of mutant RTs on RNA/DNA vs. DNA/DNA templates .
3.2 Synthesis and characterization protocols:
Properties
IUPAC Name |
2-amino-9-[(2R,4S,5S)-4-amino-5-(hydroxymethyl)oxolan-2-yl]-1H-purin-6-one | |
---|---|---|
Source | PubChem | |
URL | https://pubchem.ncbi.nlm.nih.gov | |
Description | Data deposited in or computed by PubChem | |
InChI |
InChI=1S/C10H14N6O3/c11-4-1-6(19-5(4)2-17)16-3-13-7-8(16)14-10(12)15-9(7)18/h3-6,17H,1-2,11H2,(H3,12,14,15,18)/t4-,5+,6+/m0/s1 | |
Source | PubChem | |
URL | https://pubchem.ncbi.nlm.nih.gov | |
Description | Data deposited in or computed by PubChem | |
InChI Key |
HOQBPAWPBCAUGA-KVQBGUIXSA-N | |
Source | PubChem | |
URL | https://pubchem.ncbi.nlm.nih.gov | |
Description | Data deposited in or computed by PubChem | |
Canonical SMILES |
C1C(C(OC1N2C=NC3=C2N=C(NC3=O)N)CO)N | |
Source | PubChem | |
URL | https://pubchem.ncbi.nlm.nih.gov | |
Description | Data deposited in or computed by PubChem | |
Isomeric SMILES |
C1[C@@H]([C@H](O[C@H]1N2C=NC3=C2N=C(NC3=O)N)CO)N | |
Source | PubChem | |
URL | https://pubchem.ncbi.nlm.nih.gov | |
Description | Data deposited in or computed by PubChem | |
Molecular Formula |
C10H14N6O3 | |
Source | PubChem | |
URL | https://pubchem.ncbi.nlm.nih.gov | |
Description | Data deposited in or computed by PubChem | |
DSSTOX Substance ID |
DTXSID70216535 | |
Record name | Guanosine, 3'-amino-2',3'-dideoxy- | |
Source | EPA DSSTox | |
URL | https://comptox.epa.gov/dashboard/DTXSID70216535 | |
Description | DSSTox provides a high quality public chemistry resource for supporting improved predictive toxicology. | |
Molecular Weight |
266.26 g/mol | |
Source | PubChem | |
URL | https://pubchem.ncbi.nlm.nih.gov | |
Description | Data deposited in or computed by PubChem | |
CAS No. |
66323-49-7 | |
Record name | Guanosine, 3'-amino-2',3'-dideoxy- | |
Source | ChemIDplus | |
URL | https://pubchem.ncbi.nlm.nih.gov/substance/?source=chemidplus&sourceid=0066323497 | |
Description | ChemIDplus is a free, web search system that provides access to the structure and nomenclature authority files used for the identification of chemical substances cited in National Library of Medicine (NLM) databases, including the TOXNET system. | |
Record name | Guanosine, 3'-amino-2',3'-dideoxy- | |
Source | EPA DSSTox | |
URL | https://comptox.epa.gov/dashboard/DTXSID70216535 | |
Description | DSSTox provides a high quality public chemistry resource for supporting improved predictive toxicology. | |
Retrosynthesis Analysis
AI-Powered Synthesis Planning: Our tool employs the Template_relevance Pistachio, Template_relevance Bkms_metabolic, Template_relevance Pistachio_ringbreaker, Template_relevance Reaxys, Template_relevance Reaxys_biocatalysis model, leveraging a vast database of chemical reactions to predict feasible synthetic routes.
One-Step Synthesis Focus: Specifically designed for one-step synthesis, it provides concise and direct routes for your target compounds, streamlining the synthesis process.
Accurate Predictions: Utilizing the extensive PISTACHIO, BKMS_METABOLIC, PISTACHIO_RINGBREAKER, REAXYS, REAXYS_BIOCATALYSIS database, our tool offers high-accuracy predictions, reflecting the latest in chemical research and data.
Strategy Settings
Precursor scoring | Relevance Heuristic |
---|---|
Min. plausibility | 0.01 |
Model | Template_relevance |
Template Set | Pistachio/Bkms_metabolic/Pistachio_ringbreaker/Reaxys/Reaxys_biocatalysis |
Top-N result to add to graph | 6 |
Feasible Synthetic Routes
Disclaimer and Information on In-Vitro Research Products
Please be aware that all articles and product information presented on BenchChem are intended solely for informational purposes. The products available for purchase on BenchChem are specifically designed for in-vitro studies, which are conducted outside of living organisms. In-vitro studies, derived from the Latin term "in glass," involve experiments performed in controlled laboratory settings using cells or tissues. It is important to note that these products are not categorized as medicines or drugs, and they have not received approval from the FDA for the prevention, treatment, or cure of any medical condition, ailment, or disease. We must emphasize that any form of bodily introduction of these products into humans or animals is strictly prohibited by law. It is essential to adhere to these guidelines to ensure compliance with legal and ethical standards in research and experimentation.