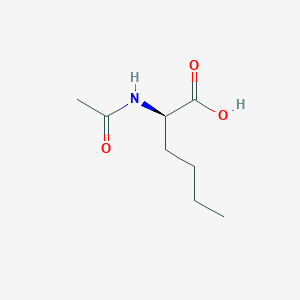
N-Acetyl-D-norleucine
Overview
Description
N-Acetyl-D-norleucine is a derivative of the amino acid D-norleucine. It is characterized by the presence of an acetyl group attached to the nitrogen atom of the amino acid. This compound is a white solid that is soluble in water and organic solvents. It is stable under acidic and neutral conditions but can hydrolyze under alkaline conditions .
Preparation Methods
Synthetic Routes and Reaction Conditions
N-Acetyl-D-norleucine can be synthesized through two primary methods:
Reaction of D-norleucine with Acetyl Chloride: This method involves the reaction of D-norleucine with acetyl chloride to form an intermediate, which is then reacted with acetic anhydride to yield this compound.
Acetylation of D-norleucine: This method involves the direct acetylation of D-norleucine using acetic anhydride in the presence of a base such as pyridine.
Industrial Production Methods
Industrial production of this compound typically involves the acetylation of D-norleucine using acetic anhydride. The reaction is carried out in a solvent such as methanol or ethanol, and the product is purified through crystallization or chromatography .
Chemical Reactions Analysis
Types of Reactions
N-Acetyl-D-norleucine undergoes various chemical reactions, including:
Hydrolysis: Under alkaline conditions, this compound can hydrolyze to form D-norleucine and acetic acid.
Oxidation: This compound can be oxidized to form corresponding oxo derivatives.
Substitution: The acetyl group can be substituted with other functional groups under appropriate conditions.
Common Reagents and Conditions
Hydrolysis: Sodium hydroxide or potassium hydroxide in aqueous solution.
Oxidation: Oxidizing agents such as potassium permanganate or hydrogen peroxide.
Substitution: Various nucleophiles in the presence of a base.
Major Products Formed
Hydrolysis: D-norleucine and acetic acid.
Oxidation: Oxo derivatives of this compound.
Substitution: Derivatives with substituted functional groups.
Scientific Research Applications
N-Acetyl-D-norleucine has several applications in scientific research:
Mechanism of Action
N-Acetyl-D-norleucine exerts its effects by being taken up into cells through specific transporters. It is believed to act as a prodrug for D-norleucine, which then enters metabolic pathways and exerts its effects through its metabolic products. The compound is taken up by organic anion transporters and monocarboxylate transporters, bypassing the rate-limiting step in the activation of leucine-mediated signaling and metabolic processes .
Comparison with Similar Compounds
Similar Compounds
N-Acetyl-D-leucine: Similar in structure but with a different side chain.
N-Acetyl-L-norleucine: The L-enantiomer of N-Acetyl-D-norleucine.
N-Acetyl-DL-norleucine: A racemic mixture of both D- and L-enantiomers.
Uniqueness
This compound is unique due to its specific stereochemistry and its ability to be taken up by specific transporters, which makes it a valuable compound for studying metabolic pathways and for potential therapeutic applications .
Properties
IUPAC Name |
(2R)-2-acetamidohexanoic acid | |
---|---|---|
Source | PubChem | |
URL | https://pubchem.ncbi.nlm.nih.gov | |
Description | Data deposited in or computed by PubChem | |
InChI |
InChI=1S/C8H15NO3/c1-3-4-5-7(8(11)12)9-6(2)10/h7H,3-5H2,1-2H3,(H,9,10)(H,11,12)/t7-/m1/s1 | |
Source | PubChem | |
URL | https://pubchem.ncbi.nlm.nih.gov | |
Description | Data deposited in or computed by PubChem | |
InChI Key |
JDMCEGLQFSOMQH-SSDOTTSWSA-N | |
Source | PubChem | |
URL | https://pubchem.ncbi.nlm.nih.gov | |
Description | Data deposited in or computed by PubChem | |
Canonical SMILES |
CCCCC(C(=O)O)NC(=O)C | |
Source | PubChem | |
URL | https://pubchem.ncbi.nlm.nih.gov | |
Description | Data deposited in or computed by PubChem | |
Isomeric SMILES |
CCCC[C@H](C(=O)O)NC(=O)C | |
Source | PubChem | |
URL | https://pubchem.ncbi.nlm.nih.gov | |
Description | Data deposited in or computed by PubChem | |
Molecular Formula |
C8H15NO3 | |
Source | PubChem | |
URL | https://pubchem.ncbi.nlm.nih.gov | |
Description | Data deposited in or computed by PubChem | |
DSSTOX Substance ID |
DTXSID401031698 | |
Record name | N-Acetyl-D-norleucine | |
Source | EPA DSSTox | |
URL | https://comptox.epa.gov/dashboard/DTXSID401031698 | |
Description | DSSTox provides a high quality public chemistry resource for supporting improved predictive toxicology. | |
Molecular Weight |
173.21 g/mol | |
Source | PubChem | |
URL | https://pubchem.ncbi.nlm.nih.gov | |
Description | Data deposited in or computed by PubChem | |
CAS No. |
54896-21-8 | |
Record name | N-Acetyl-D-norleucine | |
Source | ChemIDplus | |
URL | https://pubchem.ncbi.nlm.nih.gov/substance/?source=chemidplus&sourceid=0054896218 | |
Description | ChemIDplus is a free, web search system that provides access to the structure and nomenclature authority files used for the identification of chemical substances cited in National Library of Medicine (NLM) databases, including the TOXNET system. | |
Record name | N-Acetyl-D-norleucine | |
Source | EPA DSSTox | |
URL | https://comptox.epa.gov/dashboard/DTXSID401031698 | |
Description | DSSTox provides a high quality public chemistry resource for supporting improved predictive toxicology. | |
Record name | N-ACETYL-D-NORLEUCINE | |
Source | FDA Global Substance Registration System (GSRS) | |
URL | https://gsrs.ncats.nih.gov/ginas/app/beta/substances/99UH0ZG4JV | |
Description | The FDA Global Substance Registration System (GSRS) enables the efficient and accurate exchange of information on what substances are in regulated products. Instead of relying on names, which vary across regulatory domains, countries, and regions, the GSRS knowledge base makes it possible for substances to be defined by standardized, scientific descriptions. | |
Explanation | Unless otherwise noted, the contents of the FDA website (www.fda.gov), both text and graphics, are not copyrighted. They are in the public domain and may be republished, reprinted and otherwise used freely by anyone without the need to obtain permission from FDA. Credit to the U.S. Food and Drug Administration as the source is appreciated but not required. | |
Retrosynthesis Analysis
AI-Powered Synthesis Planning: Our tool employs the Template_relevance Pistachio, Template_relevance Bkms_metabolic, Template_relevance Pistachio_ringbreaker, Template_relevance Reaxys, Template_relevance Reaxys_biocatalysis model, leveraging a vast database of chemical reactions to predict feasible synthetic routes.
One-Step Synthesis Focus: Specifically designed for one-step synthesis, it provides concise and direct routes for your target compounds, streamlining the synthesis process.
Accurate Predictions: Utilizing the extensive PISTACHIO, BKMS_METABOLIC, PISTACHIO_RINGBREAKER, REAXYS, REAXYS_BIOCATALYSIS database, our tool offers high-accuracy predictions, reflecting the latest in chemical research and data.
Strategy Settings
Precursor scoring | Relevance Heuristic |
---|---|
Min. plausibility | 0.01 |
Model | Template_relevance |
Template Set | Pistachio/Bkms_metabolic/Pistachio_ringbreaker/Reaxys/Reaxys_biocatalysis |
Top-N result to add to graph | 6 |
Feasible Synthetic Routes
Disclaimer and Information on In-Vitro Research Products
Please be aware that all articles and product information presented on BenchChem are intended solely for informational purposes. The products available for purchase on BenchChem are specifically designed for in-vitro studies, which are conducted outside of living organisms. In-vitro studies, derived from the Latin term "in glass," involve experiments performed in controlled laboratory settings using cells or tissues. It is important to note that these products are not categorized as medicines or drugs, and they have not received approval from the FDA for the prevention, treatment, or cure of any medical condition, ailment, or disease. We must emphasize that any form of bodily introduction of these products into humans or animals is strictly prohibited by law. It is essential to adhere to these guidelines to ensure compliance with legal and ethical standards in research and experimentation.