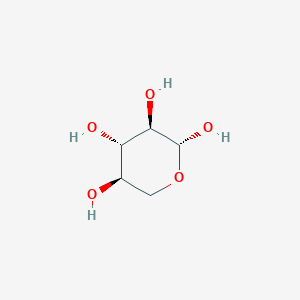
beta-D-Xylopyranose
Overview
Description
Beta-D-Xylopyranose is a cyclic monosaccharide and the pyranose form of D-xylose, a pentose sugar. Its molecular formula is C₅H₁₀O₅, with an average molecular mass of 150.130 Da and a monoisotopic mass of 150.052823 Da . The compound features a six-membered pyranose ring with four stereocenters and a β-configuration at the anomeric carbon (C1), where the hydroxyl group adopts an axial position in the chair conformation. This compound is a key structural component of xylan, a hemicellulose polysaccharide abundant in plant cell walls, and serves as a substrate for glycoside hydrolases in biomass degradation .
Preparation Methods
Synthetic Routes and Reaction Conditions
The reaction conditions typically include the use of organic solvents, controlled temperatures, and specific catalysts to ensure high yield and purity .
Industrial Production Methods
Industrial production of 2-O-DMT-Sulfonyldiethanol phosphoramidite follows similar synthetic routes but on a larger scale. The process involves rigorous quality control measures to maintain the compound’s purity and consistency. The compound is usually stored at low temperatures to preserve its stability .
Chemical Reactions Analysis
Types of Reactions
2-O-DMT-Sulfonyldiethanol phosphoramidite undergoes various chemical reactions, including:
Oxidation: This reaction involves the addition of oxygen or the removal of hydrogen, often using oxidizing agents.
Reduction: The compound can be reduced by adding hydrogen or removing oxygen, typically using reducing agents.
Substitution: This reaction involves the replacement of one functional group with another, often facilitated by specific catalysts
Common Reagents and Conditions
Common reagents used in these reactions include oxidizing agents like hydrogen peroxide, reducing agents like sodium borohydride, and catalysts like palladium. The reaction conditions vary depending on the desired outcome, but they generally involve controlled temperatures and specific solvents .
Major Products Formed
The major products formed from these reactions depend on the specific reaction pathway. For example, oxidation may yield sulfoxides or sulfones, while reduction may produce alcohols or amines .
Scientific Research Applications
Biomass Conversion and Pyrolysis
Role in Hemicellulose Modeling
β-D-Xylopyranose serves as a model structural motif for hemicellulose, which is one of the main components of lignocellulosic biomass. Recent studies have employed quantum chemical modeling to investigate the pyrolytic reactivity of β-D-xylopyranose. The thermal degradation pathways have been analyzed using advanced computational techniques, revealing insights into its potential as a precursor for biofuels like furfural .
Thermochemical Kinetics
Research indicates that the pyrolysis of β-D-xylopyranose involves complex reaction mechanisms that can be modeled to optimize biofuel production. The kinetics of these reactions have been studied extensively, with findings suggesting that functional groups attached to β-D-xylopyranose significantly influence its thermal degradation behavior . A detailed kinetic model has been developed to understand these processes better, providing valuable data for biomass modeling communities .
Antimicrobial Properties
Antimicrobial Activity Studies
Recent investigations into β-D-xylopyranosides and their derivatives have demonstrated promising antimicrobial properties against various pathogens. In particular, compounds derived from β-D-xylopyranose exhibited significant activity against fungi such as Candida albicans and bacteria including Staphylococcus aureus and Escherichia coli. These studies utilized methods like the Ames test to assess mutagenic potential, confirming that certain derivatives do not exhibit mutagenicity while maintaining antimicrobial efficacy .
Potential Therapeutic Applications
The antimicrobial activity of β-D-xylopyranosides suggests potential therapeutic applications in treating infections caused by resistant strains of bacteria. The structure-activity relationship studies conducted on these compounds indicate that modifications to the sugar moiety can enhance their biological activity, paving the way for novel antibiotic development .
Case Studies and Research Findings
Mechanism of Action
The mechanism of action of 2-O-DMT-Sulfonyldiethanol phosphoramidite involves its ability to form stable bonds with nucleic acids. This interaction allows for the precise modification and synthesis of nucleic acid sequences. The compound targets specific molecular pathways and structures, enabling researchers to manipulate genetic material with high accuracy .
Comparison with Similar Compounds
Structural Comparison with Alpha-D-Xylopyranose
Beta-D-Xylopyranose and its anomer, alpha-D-Xylopyranose, differ solely in the configuration of the hydroxyl group at C1 (axial in β, equatorial in α). This distinction influences their conformational stability and molecular interactions.
Table 1: Structural and Dynamic Properties of Anomers
Property | This compound | Alpha-D-Xylopyranose | Reference |
---|---|---|---|
Anomeric Configuration | β (axial -OH) | α (equatorial -OH) | |
Average SASA (Ų) | 157.0879 | 157.1041 | |
SASA Standard Deviation | 1.289849 | 1.154096 |
Molecular dynamics simulations reveal nearly identical solvent-accessible surface areas (SASA) for both anomers, but this compound exhibits greater structural flexibility, as indicated by its higher SASA standard deviation . This flexibility may enhance its adaptability in enzyme binding pockets.
Comparison with Other Monosaccharides
This compound shares functional similarities with hexoses like beta-D-glucopyranose and beta-D-galactopyranose but differs in ring substituents and biological roles.
Table 2: Comparison with Beta-D-Glucopyranose and Beta-D-Galactopyranose
The absence of a sixth carbon in this compound reduces steric hindrance, facilitating its incorporation into branched hemicellulose structures like arabinoxylan .
Comparison with Glycoside Derivatives
This compound forms glycosidic bonds with aglycones, yielding derivatives with distinct physicochemical properties.
Examples of Glycosides :
- Phenyl-β-D-glucopyranoside: A glucoside with a phenyl group; structural studies show that small differences (e.g., glucose vs. galactose) significantly alter intermolecular interactions .
- Isorhamnetin 3-O-[β-D-xylopyranosyl-(1→6)-β-D-glucopyranoside]: A flavonoid glycoside where xylose enhances solubility and bioavailability .
Table 3: Enzymatic Hydrolysis Rates of Xylan Derivatives
Substrate | Enzyme | Hydrolysis Rate | Reference |
---|---|---|---|
Acetylated xylan | Xylanase | Low | |
Deacetylated xylan | Xylanase | High | |
4-Nitrophenyl xylopyranoside | Xylan deacetylase | Moderate |
Enzymes like xylan deacetylases show specificity for this compound-containing substrates, with acetylation levels directly impacting hydrolysis efficiency .
Role in Polysaccharides and Enzymatic Interactions
This compound is a repeating unit in xylan, a polymer critical to plant cell wall integrity. Xylanases target β-1,4 linkages between xylose residues, but acetylation at O-2 or O-3 positions hinders hydrolysis .
Key Findings :
- Evolutionary Adaptation: Residues in xylanase substrate-binding pockets (e.g., GH11 family) show positive selection (Ka/Ks > 1), optimizing interactions with this compound .
- Biotechnological Impact: Reducing xylan acetylation by ~20% improves saccharification yields by 10–15% in engineered plants .
Physicochemical Properties and Reactivity
This compound is less soluble in water than hexoses due to its smaller size and reduced hydroxyl density. Acetylation further modulates its hydrophobicity and enzymatic accessibility .
Comparative Reactivity :
- Deoxygenation: Beta-D-Digitoxopyranose (2,6-dideoxy derivative) exhibits reduced polarity and altered bioactivity compared to this compound .
- Chromogenic Substrates: 4-Nitrophenyl derivatives of this compound enable high-throughput screening of xylanolytic enzymes .
Biological Activity
β-D-Xylopyranose, commonly referred to as β-D-xylose, is a pentose sugar that plays a crucial role in various biological processes. It is a fundamental component of hemicellulose, a polysaccharide found in plant cell walls, and has garnered attention for its potential applications in pharmaceuticals and biotechnology. This article explores the biological activity of β-D-xylopyranose, focusing on its antimicrobial properties, enzymatic interactions, and potential therapeutic applications.
Chemical Structure and Properties
β-D-Xylopyranose is characterized by its five-carbon sugar structure, which can exist in multiple anomeric forms. The β configuration is significant due to its influence on the compound's reactivity and biological interactions. The stereochemistry of β-D-xylopyranose affects its solubility, stability, and interaction with enzymes and receptors.
Antimicrobial Activity
Recent studies have highlighted the antimicrobial properties of β-D-xylopyranose derivatives. A study published in Antibiotics demonstrated that glycosides derived from β-D-xylopyranose exhibited significant antimicrobial activity against various pathogens, including Candida albicans , Staphylococcus aureus , and Escherichia coli . The minimum inhibitory concentration (MIC) values for these compounds ranged from 32 to 256 μg/mL, indicating their potential as antimicrobial agents .
Table 1: Antimicrobial Activity of β-D-Xylopyranose Derivatives
Compound | Target Pathogen | MIC (μg/mL) |
---|---|---|
Glycoside 1 | C. albicans | 64 |
Glycoside 2 | S. aureus | 32 |
Glycoside 3 | E. coli | 256 |
Glycoside 4 | C. glabrata | 128 |
Enzymatic Recognition
β-D-Xylopyranose is also recognized by various enzymes, particularly xylanases, which are crucial for the degradation of hemicellulose in plant biomass. A study on xylanase GH11 from Thermoanaerobacterium saccharolyticum revealed that this enzyme has a high catalytic efficiency (k_cat value of 34,015 s⁻¹ at pH 5.0 and 70 °C) towards beechwood xylan, suggesting that β-D-xylopyranose plays a vital role in industrial applications related to biomass conversion .
Pyrolysis and Thermal Degradation
The thermal degradation of β-D-xylopyranose has been extensively studied due to its implications in biomass energy production. Computational models have been developed to understand the pyrolytic pathways of β-D-xylopyranose, identifying key intermediates such as xylulose that lead to the formation of valuable biofuels like furfural . This research underscores the importance of β-D-xylopyranose not only as a structural component but also as a precursor for biofuel production.
Case Studies and Applications
- Antimicrobial Applications : The derivatives of β-D-xylopyranose have been explored for their potential use in treating infections caused by resistant bacterial strains. The increased activity of certain derivatives against both methicillin-resistant (MRSA) and methicillin-sensitive (MSSA) strains highlights their therapeutic promise .
- Biomass Conversion : The recognition of β-D-xylopyranose by xylanases opens avenues for its use in bioethanol production from lignocellulosic biomass. The efficient breakdown of hemicellulose into fermentable sugars could significantly enhance biofuel yields .
- Pharmaceutical Development : The structural properties of β-D-xylopyranose make it a candidate for developing new oligosaccharides with specific biological activities, potentially leading to novel therapeutic agents .
Q & A
Basic Research Questions
Q. What experimental methods are most reliable for determining the crystal structure of beta-D-xylopyranose, and how can accuracy be validated?
- Methodological Answer : X-ray crystallography is the gold standard for resolving the three-dimensional structure of this compound. For example, studies on beta-D-xylosidase DtXyl used X-ray diffraction (2.67 Å resolution) to analyze substrate binding, with validation through structural refinement software (e.g., PHENIX) and comparison to known carbohydrate geometries . Accuracy is ensured by iterative model building, electron density map analysis, and cross-validation using tools like MolProbity for steric clashes and Ramachandran plot outliers.
Q. How can this compound be synthesized and purified for enzymatic studies?
- Methodological Answer : this compound derivatives (e.g., 4-nitrophenyl beta-D-xyloside) are synthesized via glycosylation reactions using activated xylose donors (e.g., trichloroacetimidates) under anhydrous conditions. Purification involves column chromatography (silica gel or size-exclusion) and characterization via NMR (e.g., anomeric proton signals at δ 4.5–5.5 ppm) and high-resolution mass spectrometry (HRMS) . Enzymatic transxylosylation using beta-xylosidases (e.g., Aspergillus niger) can also generate xylooligosaccharides, with products isolated via charcoal column chromatography and thin-layer chromatography (TLC) .
Q. What analytical techniques are optimal for quantifying this compound in complex mixtures?
- Methodological Answer : High-performance liquid chromatography (HPLC) with refractive index (RI) or evaporative light scattering detection (ELSD) is preferred for quantification. For higher sensitivity, mass spectrometry (LC-MS/MS) in multiple reaction monitoring (MRM) mode provides specificity in biological matrices. Calibration curves using pure standards and internal normalization (e.g., xylitol as an internal standard) improve accuracy .
Advanced Research Questions
Q. How do hydrophobic residues in beta-D-xylosidases influence substrate binding and catalytic efficiency for this compound hydrolysis?
- Methodological Answer : Structural studies of DtXyl (PDB: 6YYI) revealed that non-conserved hydrophobic residues (e.g., Trp286, Phe161) form π-stacking interactions with the xylose ring, enhancing substrate affinity. Mutagenesis experiments (e.g., alanine scanning) combined with kinetic assays (Km and kcat measurements) can quantify residue contributions. Molecular dynamics simulations further assess conformational stability during catalysis .
Q. How can contradictory data on this compound hydrolysis rates under varying pH and temperature conditions be resolved?
- Methodological Answer : Contradictions often arise from differences in enzyme sources (e.g., thermophilic vs. mesophilic xylosidases) or assay buffers. Systematic reproducibility studies should control ionic strength, buffer composition (e.g., citrate vs. phosphate), and pre-incubation conditions. Statistical tools like ANOVA can identify significant variables, while isothermal titration calorimetry (ITC) provides thermodynamic insights into pH-dependent binding .
Q. What strategies are effective for engineering beta-D-xylosidase mutants with enhanced activity on this compound derivatives?
- Methodological Answer : Rational design using structural data (e.g., active-site topology from 8YYN) guides residue substitutions to optimize substrate docking. Directed evolution via error-prone PCR and high-throughput screening (HTS) in E. coli libraries can isolate mutants with improved kcat/Km. Activity assays using chromogenic substrates (e.g., pNP-beta-D-xylopyranoside) enable rapid kinetic profiling .
Q. How does this compound participate in transxylosylation reactions, and what factors dictate product specificity?
- Methodological Answer : Transxylosylation by beta-xylosidases involves a retaining mechanism where the enzyme transfers the xylosyl moiety to acceptor molecules (e.g., D-mannose). Product specificity is controlled by the enzyme’s subsite architecture and acceptor binding affinity. NMR and methylation analysis identify glycosidic linkages (e.g., β-(1→3) vs. β-(1→4)), while molecular docking simulations predict acceptor orientation in the active site .
Q. What are the challenges in modeling this compound interactions in polysaccharides like xylan, and how can they be addressed?
- Methodological Answer : Xylan’s heterogeneity (e.g., arabinose side chains) complicates modeling. Solid-state NMR and small-angle X-ray scattering (SAXS) provide insights into polymer conformation. Molecular dynamics simulations using force fields (e.g., GLYCAM06) must account for solvation effects and glycosidic linkage flexibility. Experimental validation via enzymatic hydrolysis profiles (e.g., using endoxylanases) confirms model accuracy .
Q. Methodological Design Considerations
- Structural Validation : Always cross-validate crystallographic data with orthogonal techniques (e.g., NMR for solution-state conformation) to avoid overfitting .
- Enzyme Assays : Include negative controls (e.g., heat-inactivated enzymes) and substrate blanks to account for non-enzymatic hydrolysis .
- Data Reproducibility : Document buffer compositions, enzyme lots, and instrument calibration protocols to minimize inter-lab variability .
Properties
CAS No. |
108783-02-4 |
---|---|
Molecular Formula |
C34H45N2O7PS |
Molecular Weight |
656.8 g/mol |
IUPAC Name |
3-[2-[2-[bis(4-methoxyphenyl)-phenylmethoxy]ethylsulfonyl]ethoxy-[di(propan-2-yl)amino]phosphanyl]oxypropanenitrile |
InChI |
InChI=1S/C34H45N2O7PS/c1-27(2)36(28(3)4)44(42-22-10-21-35)43-24-26-45(37,38)25-23-41-34(29-11-8-7-9-12-29,30-13-17-32(39-5)18-14-30)31-15-19-33(40-6)20-16-31/h7-9,11-20,27-28H,10,22-26H2,1-6H3 |
InChI Key |
RJDYWMSSNMDIOO-UHFFFAOYSA-N |
SMILES |
C1C(C(C(C(O1)O)O)O)O |
Isomeric SMILES |
C1[C@H]([C@@H]([C@H]([C@@H](O1)O)O)O)O |
Canonical SMILES |
CC(C)N(C(C)C)P(OCCC#N)OCCS(=O)(=O)CCOC(C1=CC=CC=C1)(C2=CC=C(C=C2)OC)C3=CC=C(C=C3)OC |
Key on ui other cas no. |
2460-44-8 |
Origin of Product |
United States |
Retrosynthesis Analysis
AI-Powered Synthesis Planning: Our tool employs the Template_relevance Pistachio, Template_relevance Bkms_metabolic, Template_relevance Pistachio_ringbreaker, Template_relevance Reaxys, Template_relevance Reaxys_biocatalysis model, leveraging a vast database of chemical reactions to predict feasible synthetic routes.
One-Step Synthesis Focus: Specifically designed for one-step synthesis, it provides concise and direct routes for your target compounds, streamlining the synthesis process.
Accurate Predictions: Utilizing the extensive PISTACHIO, BKMS_METABOLIC, PISTACHIO_RINGBREAKER, REAXYS, REAXYS_BIOCATALYSIS database, our tool offers high-accuracy predictions, reflecting the latest in chemical research and data.
Strategy Settings
Precursor scoring | Relevance Heuristic |
---|---|
Min. plausibility | 0.01 |
Model | Template_relevance |
Template Set | Pistachio/Bkms_metabolic/Pistachio_ringbreaker/Reaxys/Reaxys_biocatalysis |
Top-N result to add to graph | 6 |
Feasible Synthetic Routes
Disclaimer and Information on In-Vitro Research Products
Please be aware that all articles and product information presented on BenchChem are intended solely for informational purposes. The products available for purchase on BenchChem are specifically designed for in-vitro studies, which are conducted outside of living organisms. In-vitro studies, derived from the Latin term "in glass," involve experiments performed in controlled laboratory settings using cells or tissues. It is important to note that these products are not categorized as medicines or drugs, and they have not received approval from the FDA for the prevention, treatment, or cure of any medical condition, ailment, or disease. We must emphasize that any form of bodily introduction of these products into humans or animals is strictly prohibited by law. It is essential to adhere to these guidelines to ensure compliance with legal and ethical standards in research and experimentation.