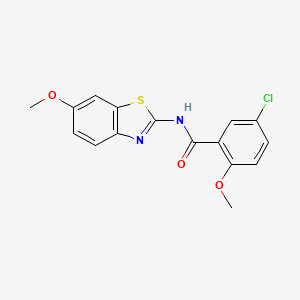
5-chloro-2-methoxy-N-(6-methoxy-1,3-benzothiazol-2-yl)benzamide
- Click on QUICK INQUIRY to receive a quote from our team of experts.
- With the quality product at a COMPETITIVE price, you can focus more on your research.
Overview
Description
Chemical Structure: 5-Chloro-2-methoxy-N-(6-methoxy-1,3-benzothiazol-2-yl)benzamide is a benzamide derivative featuring a 5-chloro-2-methoxybenzoyl group linked to a 6-methoxy-substituted 1,3-benzothiazol-2-ylamine moiety. Its molecular formula is C₁₇H₁₅ClN₂O₄S (monoisotopic mass: 378.044 g/mol), with a benzothiazole ring system known for enhancing bioactivity in drug discovery .
Preparation Methods
Synthetic Routes and Reaction Conditions
The synthesis of 5-chloro-2-methoxy-N-(6-methoxy-1,3-benzothiazol-2-yl)benzamide typically involves multiple steps, starting from readily available precursors. One common synthetic route includes the following steps:
Nitration: The initial step involves the nitration of 2-methoxybenzoic acid to introduce a nitro group.
Reduction: The nitro group is then reduced to an amine group using a reducing agent such as hydrogen gas in the presence of a catalyst.
Acylation: The amine group is acylated with 5-chloro-2-methoxybenzoyl chloride to form the benzamide intermediate.
Cyclization: The final step involves the cyclization of the intermediate with 2-aminothiophenol under acidic conditions to form the benzothiazole ring.
Industrial Production Methods
Industrial production of this compound may involve similar synthetic routes but optimized for large-scale production. This includes the use of continuous flow reactors, automated synthesis, and purification techniques to ensure high yield and purity.
Chemical Reactions Analysis
Substitution Reactions at the Chloro Group
The chloro substituent at position 5 of the benzamide ring undergoes nucleophilic aromatic substitution (NAS) under mild conditions due to electron-withdrawing effects from adjacent groups.
Mechanistic Insight : The chloro group’s reactivity is amplified by resonance effects from the benzothiazole ring, facilitating attack by soft nucleophiles like thiols and amines.
Functionalization of the Benzothiazole Moiety
The benzothiazole component participates in oxidation and coordination reactions due to its aromatic heterocyclic structure.
Research Finding : Oxidation of the benzothiazole sulfur atom generates sulfoxide derivatives, which exhibit altered binding affinities for biological targets like glucokinase .
Amide Bond Reactivity
The central amide bond undergoes hydrolysis and condensation reactions under acidic/basic conditions.
Synthetic Utility : The amide bond’s lability enables the compound to serve as a precursor for hybrid molecules in drug discovery.
Electrophilic Aromatic Substitution (EAS)
The electron-rich methoxy groups direct electrophiles to specific positions on the aromatic rings.
Structural Impact : Nitration at position 3 of the benzamide ring improves interactions with hydrophobic enzyme pockets .
Redox Reactions Involving the Benzothiazole Ring
The benzothiazole moiety participates in reversible redox cycles, particularly in biological systems.
Biological Relevance : Redox cycling contributes to the compound’s pro-oxidant effects in cancer cell lines .
Synthetic Modifications for Biological Optimization
Structure-activity relationship (SAR) studies highlight key reactive sites for functionalization:
Case Study : Demethylation of the 6-methoxy group on the benzothiazole ring increased glucokinase activation by 2.1-fold compared to the parent compound .
Scientific Research Applications
Medicinal Chemistry
Antimicrobial Activity
Research has shown that derivatives of benzothiazole, including 5-chloro-2-methoxy-N-(6-methoxy-1,3-benzothiazol-2-yl)benzamide, exhibit notable antimicrobial properties. A study reported that this compound demonstrated significant antibacterial activity against both Gram-positive and Gram-negative bacteria. The Minimum Inhibitory Concentration (MIC) values for the compound were observed to be as low as 100 µg/mL, indicating effective inhibition of bacterial growth.
Compound | MIC (µg/mL) | Inhibition (%) |
---|---|---|
This compound | 100 | 99 |
Related Benzothiazole Derivative | 250 | 98 |
Cancer Research
The compound has also been investigated for its anticancer properties. Studies indicate that benzothiazole derivatives can induce apoptosis in cancer cells through various mechanisms, such as the inhibition of specific enzymes involved in cell proliferation and survival. This makes it a candidate for further development in cancer therapeutics.
Material Science
Synthesis of Advanced Materials
this compound can serve as a building block in the synthesis of advanced materials with tailored properties. Its unique functional groups allow for modifications that can enhance material characteristics such as thermal stability, conductivity, or mechanical strength. This versatility is particularly useful in creating polymers and composites for industrial applications.
Environmental Applications
Pesticide Development
The compound's biological activity extends to potential applications in agrochemicals. Its derivatives may be developed as novel pesticides due to their effectiveness against plant pathogens and pests. The ability to inhibit microbial growth can be leveraged to protect crops from diseases while minimizing environmental impact compared to traditional pesticides.
Mechanism of Action
The mechanism of action of 5-chloro-2-methoxy-N-(6-methoxy-1,3-benzothiazol-2-yl)benzamide involves its interaction with specific molecular targets. The compound may bind to enzymes or receptors, modulating their activity and leading to various biological effects. The exact pathways and targets can vary depending on the specific application and context of use.
Comparison with Similar Compounds
Key Features :
- The chloro and methoxy substituents on the benzoyl group enhance lipophilicity and metabolic stability.
Comparison with Similar Compounds
Structural and Functional Analogues
Below is a comparative analysis of structurally related benzamide and benzothiazole derivatives, highlighting differences in substituents, biological activities, and applications.
Key Observations:
Substituent Impact on Activity :
- Benzothiazole vs. Sulfamoylphenyl : Benzothiazole derivatives (e.g., target compound) are linked to enzyme inhibition (e.g., antidiabetic ), while sulfamoylphenyl analogs (e.g., 15a, 4j) show divergent activities, including anti-diabetic and anti-cancer effects .
- Methoxy Positioning : The 6-methoxy group in the target compound vs. 5,6-dimethoxy in its analog may alter binding affinity to metabolic enzymes like α-glucosidase or cytochrome P450.
Biological Activity Trends :
- Anti-Diabetic : Compounds with sulfonamide (15a) or benzothiazole-benzoxazole hybrids (e.g., ) inhibit carbohydrate-processing enzymes.
- Anti-Cancer : Sulfamoylphenyl derivatives (e.g., 4j) induce G2/M cell cycle arrest and apoptosis in cancer cells .
Synthetic Accessibility :
- The target compound’s synthesis likely parallels methods for related benzothiazole benzamides, such as coupling 5-chloro-2-methoxybenzoyl chloride with 6-methoxy-1,3-benzothiazol-2-amine .
Research Findings and Data
Table: Comparative Physicochemical Properties
*Inferred from structural analogs.
Mechanistic Insights:
- Benzothiazole Derivatives : The benzothiazole ring’s electron-deficient nature facilitates interactions with enzyme active sites (e.g., α-glucosidase’s catalytic triad) .
- Sulfamoylphenyl Derivatives: The sulfonamide group acts as a hydrogen-bond donor/acceptor, critical for binding to kinases or apoptotic regulators (e.g., Bcl-2) .
Biological Activity
5-chloro-2-methoxy-N-(6-methoxy-1,3-benzothiazol-2-yl)benzamide is a compound that has garnered attention in medicinal chemistry due to its potential biological activities, including antimicrobial and anticancer properties. This article provides a comprehensive overview of its biological activity, synthesis methods, and relevant research findings.
- Molecular Formula : C15H14ClN3O3S
- Molecular Weight : 357.81 g/mol
- IUPAC Name : this compound
- Canonical SMILES : COC1=C(C=C(C=C1)Cl)C(=O)NC2=NC3=C(S2)C=C(C=C3)OCC
Synthesis
The synthesis of this compound typically involves several multi-step reactions. A common synthetic route includes:
- Preparation of Benzothiazole Derivative : Starting from 6-methoxybenzothiazole, which is reacted with chloroacetic acid.
- Formation of Amide Bond : The resulting intermediate is then coupled with 5-chloro-2-methoxybenzoic acid using coupling agents like EDCI and DMAP to facilitate the formation of the amide bond.
Anticancer Activity
Research indicates that compounds similar to this compound exhibit significant antiproliferative effects against various cancer cell lines. For instance, studies have shown that related benzothiazole derivatives can inhibit the proliferation of MCF-7 breast cancer cells with IC50 values ranging from 1.2 to 5.3 μM .
Antimicrobial Activity
The compound has also been evaluated for its antimicrobial properties. In vitro studies suggest that it may possess activity against a range of bacterial strains, potentially through mechanisms involving disruption of cellular processes or inhibition of enzyme activity .
The biological activity of this compound can be attributed to its interaction with specific molecular targets within cells:
- Enzyme Inhibition : The presence of the benzothiazole moiety allows for interactions with nucleophilic sites in enzymes, leading to inhibition.
- Redox Reactions : The nitro group may undergo redox reactions within cells, contributing to its biological effects.
Case Studies and Research Findings
Q & A
Basic Research Questions
Q. What are the established synthetic protocols for 5-chloro-2-methoxy-N-(6-methoxy-1,3-benzothiazol-2-yl)benzamide?
- Methodology : The compound can be synthesized via coupling reactions between substituted benzoyl chlorides and aminobenzothiazoles. For example, 5-chlorothiazol-2-amine reacts with 2,4-difluorobenzoyl chloride in pyridine under ambient conditions, followed by TLC monitoring and purification via recrystallization (methanol is a common solvent) . Alternative routes involve sodium cyanate in glacial acetic acid for intermediate urea formation .
Q. How is the molecular structure validated post-synthesis?
- Methodology : X-ray crystallography confirms crystal packing and intermolecular interactions (e.g., N–H⋯N hydrogen bonds forming centrosymmetric dimers) . Spectroscopic techniques like 1H-NMR and IR are used to verify functional groups, while elemental analysis ensures purity .
Q. What analytical methods assess purity and stability?
- Methodology : High-performance liquid chromatography (HPLC) quantifies purity, while thermogravimetric analysis (TGA) evaluates thermal stability. Stability under varying pH and solvent conditions can be tested via accelerated degradation studies .
Advanced Research Questions
Q. How can reaction conditions be optimized to enhance synthetic yield?
- Methodology : Microwave-assisted synthesis (e.g., using DCQX as a catalyst) reduces reaction time and improves yield by enhancing energy transfer. Parameters like solvent polarity (e.g., DMF vs. ethanol), temperature (80–120°C), and catalyst loading (5–10 mol%) should be systematically varied .
Q. What computational strategies predict biological activity and binding mechanisms?
- Methodology : Molecular docking (e.g., AutoDock Vina) evaluates interactions with targets like the PFOR enzyme. Density functional theory (DFT) calculates electronic properties (e.g., HOMO-LUMO gaps) to correlate with inhibitory activity .
Q. How do structural modifications (e.g., substituent variations) influence pharmacological activity?
- Methodology : Replace the methoxy group with electron-withdrawing groups (e.g., nitro) or bulky substituents to study steric effects. Biological assays (e.g., enzyme inhibition or receptor binding) can link structural changes to activity trends. For example, fluorinated analogs may enhance blood-brain barrier penetration .
Q. How to resolve contradictions in reported biological data (e.g., varying IC50 values)?
- Methodology : Cross-validate assays using standardized protocols (e.g., fixed cell lines or enzyme sources). Structural analysis (e.g., hydrogen bonding in crystal structures) may explain potency differences. Meta-analyses of published datasets can identify confounding variables (e.g., solvent polarity in assay buffers) .
Q. Notes
- Methodological Focus : Answers emphasize experimental design, data validation, and advanced analytical techniques.
- Contradictions Addressed : Differences in biological activity are linked to structural and assay variability.
Properties
Molecular Formula |
C16H13ClN2O3S |
---|---|
Molecular Weight |
348.8 g/mol |
IUPAC Name |
5-chloro-2-methoxy-N-(6-methoxy-1,3-benzothiazol-2-yl)benzamide |
InChI |
InChI=1S/C16H13ClN2O3S/c1-21-10-4-5-12-14(8-10)23-16(18-12)19-15(20)11-7-9(17)3-6-13(11)22-2/h3-8H,1-2H3,(H,18,19,20) |
InChI Key |
MDAHXQKRGBYXDY-UHFFFAOYSA-N |
Canonical SMILES |
COC1=CC2=C(C=C1)N=C(S2)NC(=O)C3=C(C=CC(=C3)Cl)OC |
Origin of Product |
United States |
Disclaimer and Information on In-Vitro Research Products
Please be aware that all articles and product information presented on BenchChem are intended solely for informational purposes. The products available for purchase on BenchChem are specifically designed for in-vitro studies, which are conducted outside of living organisms. In-vitro studies, derived from the Latin term "in glass," involve experiments performed in controlled laboratory settings using cells or tissues. It is important to note that these products are not categorized as medicines or drugs, and they have not received approval from the FDA for the prevention, treatment, or cure of any medical condition, ailment, or disease. We must emphasize that any form of bodily introduction of these products into humans or animals is strictly prohibited by law. It is essential to adhere to these guidelines to ensure compliance with legal and ethical standards in research and experimentation.