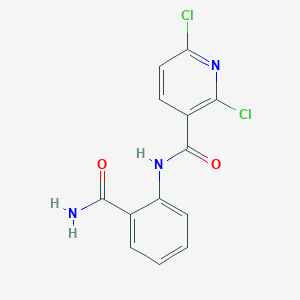
N-(2-carbamoylphenyl)-2,6-dichloropyridine-3-carboxamide
- Click on QUICK INQUIRY to receive a quote from our team of experts.
- With the quality product at a COMPETITIVE price, you can focus more on your research.
Overview
Description
N-(2-carbamoylphenyl)-2,6-dichloropyridine-3-carboxamide is a chemical compound that has garnered attention in various fields of scientific research. This compound is characterized by its unique structure, which includes a pyridine ring substituted with two chlorine atoms and a carboxamide group attached to a phenyl ring with a carbamoyl group. Its distinct chemical properties make it a valuable subject for studies in chemistry, biology, medicine, and industry.
Preparation Methods
Synthetic Routes and Reaction Conditions
The synthesis of N-(2-carbamoylphenyl)-2,6-dichloropyridine-3-carboxamide typically involves multiple steps. One common method starts with the preparation of the key intermediate, 2,6-dichloropyridine-3-carboxylic acid. This intermediate is then reacted with 2-aminobenzamide under specific conditions to form the target compound. The reaction is usually carried out in the presence of a coupling agent such as N,N’-dicyclohexylcarbodiimide (DCC) and a catalyst like 4-dimethylaminopyridine (DMAP) in an organic solvent such as dichloromethane .
Industrial Production Methods
In an industrial setting, the production of this compound can be scaled up by optimizing the reaction conditions to ensure high yield and purity. This may involve the use of continuous flow reactors, which allow for better control of reaction parameters such as temperature, pressure, and reaction time. Additionally, purification techniques such as recrystallization and chromatography are employed to obtain the final product with the desired specifications .
Chemical Reactions Analysis
Types of Reactions
N-(2-carbamoylphenyl)-2,6-dichloropyridine-3-carboxamide undergoes various chemical reactions, including:
Oxidation: The compound can be oxidized using oxidizing agents such as potassium permanganate or hydrogen peroxide.
Reduction: Reduction reactions can be carried out using reducing agents like lithium aluminum hydride or sodium borohydride.
Substitution: The chlorine atoms on the pyridine ring can be substituted with other functional groups through nucleophilic substitution reactions.
Common Reagents and Conditions
Oxidation: Potassium permanganate in an acidic medium.
Reduction: Lithium aluminum hydride in anhydrous ether.
Substitution: Sodium methoxide in methanol for nucleophilic substitution.
Major Products Formed
Oxidation: Formation of carboxylic acids or ketones.
Reduction: Formation of amines or alcohols.
Substitution: Formation of various substituted pyridine derivatives.
Scientific Research Applications
N-(2-carbamoylphenyl)-2,6-dichloropyridine-3-carboxamide has a wide range of applications in scientific research:
Chemistry: Used as a building block for the synthesis of more complex molecules.
Biology: Studied for its potential as an enzyme inhibitor or receptor ligand.
Medicine: Investigated for its therapeutic potential in treating various diseases.
Industry: Utilized in the development of new materials and chemical processes.
Mechanism of Action
The mechanism of action of N-(2-carbamoylphenyl)-2,6-dichloropyridine-3-carboxamide involves its interaction with specific molecular targets. The compound can bind to enzymes or receptors, inhibiting their activity and thereby modulating biological pathways. For example, it may inhibit the activity of certain kinases or proteases, leading to altered cellular signaling and physiological responses .
Comparison with Similar Compounds
Similar Compounds
- N-(2-carbamoylphenyl)-3,4-dichloro-1,2-thiazole-5-carboxamide
- N-(2-carbamoylphenyl)-1-(6-cyclopropylpyridazin-3-yl)piperidine-4-carboxamide
Uniqueness
N-(2-carbamoylphenyl)-2,6-dichloropyridine-3-carboxamide stands out due to its specific substitution pattern on the pyridine ring, which imparts unique chemical and biological properties. This makes it a valuable compound for targeted research and development in various scientific fields .
Properties
Molecular Formula |
C13H9Cl2N3O2 |
---|---|
Molecular Weight |
310.13 g/mol |
IUPAC Name |
N-(2-carbamoylphenyl)-2,6-dichloropyridine-3-carboxamide |
InChI |
InChI=1S/C13H9Cl2N3O2/c14-10-6-5-8(11(15)18-10)13(20)17-9-4-2-1-3-7(9)12(16)19/h1-6H,(H2,16,19)(H,17,20) |
InChI Key |
XUBVCVJJAVRWOH-UHFFFAOYSA-N |
Canonical SMILES |
C1=CC=C(C(=C1)C(=O)N)NC(=O)C2=C(N=C(C=C2)Cl)Cl |
Origin of Product |
United States |
Disclaimer and Information on In-Vitro Research Products
Please be aware that all articles and product information presented on BenchChem are intended solely for informational purposes. The products available for purchase on BenchChem are specifically designed for in-vitro studies, which are conducted outside of living organisms. In-vitro studies, derived from the Latin term "in glass," involve experiments performed in controlled laboratory settings using cells or tissues. It is important to note that these products are not categorized as medicines or drugs, and they have not received approval from the FDA for the prevention, treatment, or cure of any medical condition, ailment, or disease. We must emphasize that any form of bodily introduction of these products into humans or animals is strictly prohibited by law. It is essential to adhere to these guidelines to ensure compliance with legal and ethical standards in research and experimentation.