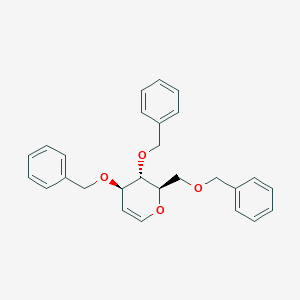
Tri-O-benzyl-D-glucal
Overview
Description
Tri-O-benzyl-D-glucal is a significant compound in the field of synthetic carbohydrate chemistry. It is a derivative of D-glucal, characterized by the presence of three benzyl groups attached to the oxygen atoms at positions 3, 4, and 6 of the glucal molecule. This compound is widely used as a building block for the synthesis of oligosaccharides, which are essential in various biological and chemical applications .
Mechanism of Action
Target of Action
Tri-O-benzyl-D-glucal, also known as 3,4,6-Tri-O-benzyl-D-glucal, is primarily used as a chiral intermediate . It is a benzyl protected, 2,3 unsaturated glucal . The compound’s primary targets are the biochemical reactions that require a chiral intermediate, particularly in the synthesis of oligosaccharides .
Mode of Action
The mode of action of this compound is based on its chemical structure. The compound has a C2-C3 double bond in the pyranose ring . This double bond can be modified via a variety of reactions, including hydrogenation, oxidation, and hydroxylation . These reactions allow the compound to interact with its targets and induce changes in the biochemical processes.
Biochemical Pathways
This compound is involved in the synthesis of oligosaccharides . Oligosaccharides are carbohydrates that consist of a small number of monosaccharides (or sugars) linked together. They play crucial roles in various biological processes, including cell-cell recognition and interaction, immune response, and infection mechanisms. The compound serves as a building block in both solid-phase and solution-phase synthesis of these oligosaccharides .
Result of Action
The primary result of this compound’s action is the production of oligosaccharides . As a chiral intermediate, it contributes to the structural complexity of the resulting oligosaccharides . These oligosaccharides can then participate in various biological processes, depending on their specific structures.
Action Environment
The action of this compound is influenced by various environmental factors. For instance, the compound is hygroscopic and should be stored in a dry and well-ventilated place . It is also insoluble in water but easily soluble in CHCl3 . These properties can affect the compound’s stability, efficacy, and the types of reactions it can participate in.
Preparation Methods
Synthetic Routes and Reaction Conditions: Tri-O-benzyl-D-glucal can be synthesized through the benzylation of D-glucal. The process typically involves the reaction of D-glucal with benzyl chloride in the presence of a base such as sodium hydride or potassium carbonate. The reaction is carried out in an aprotic solvent like dimethylformamide or tetrahydrofuran under reflux conditions .
Industrial Production Methods: In an industrial setting, the synthesis of this compound follows similar principles but on a larger scale. The reaction conditions are optimized to ensure high yield and purity. The use of continuous flow reactors and automated systems can enhance the efficiency and scalability of the production process .
Chemical Reactions Analysis
Types of Reactions: Tri-O-benzyl-D-glucal undergoes various chemical reactions, including:
Oxidation: The compound can be oxidized to form corresponding aldehydes or ketones.
Reduction: Reduction reactions can convert it into alcohols.
Substitution: It can undergo nucleophilic substitution reactions where the benzyl groups are replaced by other functional groups.
Common Reagents and Conditions:
Oxidation: Reagents like pyridinium chlorochromate or potassium permanganate are commonly used.
Reduction: Sodium borohydride or lithium aluminum hydride are typical reducing agents.
Substitution: Nucleophiles such as thiols or amines can be used under basic conditions.
Major Products: The major products formed from these reactions depend on the specific reagents and conditions used. For example, oxidation can yield benzyl-protected glucosides, while reduction can produce benzyl-protected glucitols .
Scientific Research Applications
Tri-O-benzyl-D-glucal is extensively used in scientific research, particularly in:
Chemistry: As a building block for the synthesis of complex oligosaccharides and glycoconjugates.
Biology: In the study of carbohydrate-protein interactions and the development of glycomimetics.
Medicine: For the synthesis of glycosylated drugs and vaccine candidates.
Industry: In the production of specialty chemicals and as intermediates in organic synthesis
Comparison with Similar Compounds
Tri-O-acetyl-D-glucal: Another derivative of D-glucal with acetyl groups instead of benzyl groups.
Tri-O-benzoyl-D-galactal: A similar compound with benzoyl groups and a galactal backbone.
Uniqueness: Tri-O-benzyl-D-glucal is unique due to its high reactivity and selectivity in glycosylation reactions. The benzyl groups provide stability and protection during synthesis, making it a preferred choice for complex carbohydrate synthesis .
Properties
IUPAC Name |
(2R,3S,4R)-3,4-bis(phenylmethoxy)-2-(phenylmethoxymethyl)-3,4-dihydro-2H-pyran | |
---|---|---|
Source | PubChem | |
URL | https://pubchem.ncbi.nlm.nih.gov | |
Description | Data deposited in or computed by PubChem | |
InChI |
InChI=1S/C27H28O4/c1-4-10-22(11-5-1)18-28-21-26-27(31-20-24-14-8-3-9-15-24)25(16-17-29-26)30-19-23-12-6-2-7-13-23/h1-17,25-27H,18-21H2/t25-,26-,27+/m1/s1 | |
Source | PubChem | |
URL | https://pubchem.ncbi.nlm.nih.gov | |
Description | Data deposited in or computed by PubChem | |
InChI Key |
MXYLLYBWXIUMIT-PFBJBMPXSA-N | |
Source | PubChem | |
URL | https://pubchem.ncbi.nlm.nih.gov | |
Description | Data deposited in or computed by PubChem | |
Canonical SMILES |
C1=CC=C(C=C1)COCC2C(C(C=CO2)OCC3=CC=CC=C3)OCC4=CC=CC=C4 | |
Source | PubChem | |
URL | https://pubchem.ncbi.nlm.nih.gov | |
Description | Data deposited in or computed by PubChem | |
Isomeric SMILES |
C1=CC=C(C=C1)COC[C@@H]2[C@H]([C@@H](C=CO2)OCC3=CC=CC=C3)OCC4=CC=CC=C4 | |
Source | PubChem | |
URL | https://pubchem.ncbi.nlm.nih.gov | |
Description | Data deposited in or computed by PubChem | |
Molecular Formula |
C27H28O4 | |
Source | PubChem | |
URL | https://pubchem.ncbi.nlm.nih.gov | |
Description | Data deposited in or computed by PubChem | |
DSSTOX Substance ID |
DTXSID70471566 | |
Record name | Tri-O-benzyl-D-glucal | |
Source | EPA DSSTox | |
URL | https://comptox.epa.gov/dashboard/DTXSID70471566 | |
Description | DSSTox provides a high quality public chemistry resource for supporting improved predictive toxicology. | |
Molecular Weight |
416.5 g/mol | |
Source | PubChem | |
URL | https://pubchem.ncbi.nlm.nih.gov | |
Description | Data deposited in or computed by PubChem | |
CAS No. |
55628-54-1 | |
Record name | Tri-O-benzyl-D-glucal | |
Source | EPA DSSTox | |
URL | https://comptox.epa.gov/dashboard/DTXSID70471566 | |
Description | DSSTox provides a high quality public chemistry resource for supporting improved predictive toxicology. | |
Retrosynthesis Analysis
AI-Powered Synthesis Planning: Our tool employs the Template_relevance Pistachio, Template_relevance Bkms_metabolic, Template_relevance Pistachio_ringbreaker, Template_relevance Reaxys, Template_relevance Reaxys_biocatalysis model, leveraging a vast database of chemical reactions to predict feasible synthetic routes.
One-Step Synthesis Focus: Specifically designed for one-step synthesis, it provides concise and direct routes for your target compounds, streamlining the synthesis process.
Accurate Predictions: Utilizing the extensive PISTACHIO, BKMS_METABOLIC, PISTACHIO_RINGBREAKER, REAXYS, REAXYS_BIOCATALYSIS database, our tool offers high-accuracy predictions, reflecting the latest in chemical research and data.
Strategy Settings
Precursor scoring | Relevance Heuristic |
---|---|
Min. plausibility | 0.01 |
Model | Template_relevance |
Template Set | Pistachio/Bkms_metabolic/Pistachio_ringbreaker/Reaxys/Reaxys_biocatalysis |
Top-N result to add to graph | 6 |
Feasible Synthetic Routes
Q1: What is the molecular formula and weight of 3,4,6-Tri-O-benzyl-D-glucal?
A1: 3,4,6-Tri-O-benzyl-D-glucal has the molecular formula C27H28O4 and a molecular weight of 416.51 g/mol. []
Q2: What is the significance of the twist-boat conformation of the glucal moiety in 3,4,6-Tri-O-benzyl-D-glucal?
A2: The twist-boat conformation of the glucal moiety, as revealed by X-ray crystallography, plays a crucial role in its reactivity. This conformation influences the stereoselectivity of reactions involving the double bond, particularly in electrophilic additions and glycosylation reactions. []
Q3: How can NMR spectroscopy be used to characterize 3,4,6-Tri-O-benzyl-D-glucal?
A3: NMR spectroscopy, especially 1H and 13C NMR, provides valuable information about the structure of 3,4,6-Tri-O-benzyl-D-glucal. The chemical shifts and coupling constants of the protons and carbons in the molecule can be used to determine its structure and conformation. Lanthanide shift reagents can further enhance NMR analysis by inducing predictable changes in chemical shifts, aiding in signal assignment and structural elucidation. []
Q4: What is the Ferrier rearrangement and how is 3,4,6-Tri-O-benzyl-D-glucal utilized in this reaction?
A4: The Ferrier rearrangement is a useful reaction in carbohydrate chemistry that involves the rearrangement of glycals (like 3,4,6-Tri-O-benzyl-D-glucal) to 2,3-unsaturated glycosides. Various catalysts, including Lewis acids like FeCl3/C [], H2SO4-SiO2 [, ], and BiCl3 [], can be employed to facilitate this transformation. This reaction is particularly useful for synthesizing complex oligosaccharides and glycoconjugates.
Q5: Can you elaborate on the use of triphenylphosphane hydrobromide with 3,4,6-Tri-O-benzyl-D-glucal for C-N bond formation?
A5: Triphenylphosphane hydrobromide acts as a catalyst, activating 3,4,6-Tri-O-benzyl-D-glucal for nucleophilic attack. This method facilitates direct C-N bond formation with various N-nucleophiles, leading to the synthesis of 2-deoxy-N-glycosides with high α-stereoselectivity. This approach expands the synthetic utility of 3,4,6-Tri-O-benzyl-D-glucal for creating diverse glycoconjugates. []
Q6: How can 3,4,6-Tri-O-benzyl-D-glucal be utilized for the synthesis of 2-deoxy-S-glycosides?
A6: 3,4,6-Tri-O-benzyl-D-glucal reacts with various thiols in the presence of triphenylphosphane hydrobromide, resulting in the formation of 2-deoxy-S-glycosides. The reaction typically exhibits high α-stereoselectivity, especially with sterically demanding thiols. []
Q7: What are some examples of complex molecules synthesized using 3,4,6-Tri-O-benzyl-D-glucal as a starting material?
A7: Researchers have successfully employed 3,4,6-Tri-O-benzyl-D-glucal in the synthesis of various complex molecules, showcasing its versatility. Some notable examples include:
- Aminocyclitols, (-)-Conduramine F-4, and Polyhydroxyaminoazepanes: A 'diversity-oriented' approach starting from 3,4,6-Tri-O-benzyl-D-glucal provided access to these bioactive compounds. Key transformations included a selective deprotection strategy, LiAlH4-mediated reduction, and McMurry coupling. []
- Sphinganine and Safingol: Chiron approaches utilizing 3,4,6-Tri-O-benzyl-D-glucal enabled the stereoselective synthesis of these important sphingoid bases. Key steps involved Mitsunobu reactions and olefin cross-metathesis. []
- Steviamine Analogues: Two new stereoisomers of steviamine were synthesized from 3,4,6-Tri-O-benzyl-D-glucal. The synthesis featured a modified Julia olefination as a key step and led to the discovery of a selective α-galactosidase inhibitor. []
Q8: What are some of the challenges associated with using 3,4,6-Tri-O-benzyl-D-glucal in synthesis?
A8: While 3,4,6-Tri-O-benzyl-D-glucal offers a versatile platform for carbohydrate synthesis, some challenges exist.
Q9: How does the stereochemistry at C-4 of 3,4,6-Tri-O-benzyl-D-glucal affect its reactivity?
A9: The stereochemistry at C-4 significantly influences the stereochemical outcome of reactions involving 3,4,6-Tri-O-benzyl-D-glucal. For instance, in bromination reactions, the presence or absence of substituents at C-4 and C-5 dictates whether the reaction proceeds through an open bromo oxocarbenium ion or a bridged intermediate, resulting in different diastereomeric ratios of the product dibromides. []
Q10: What is the role of substituents in the stereoselective reactions of 3,4,6-Tri-O-benzyl-D-glucal?
A10: Substituents on the 3,4,6-Tri-O-benzyl-D-glucal ring system can significantly influence the stereochemical outcome of reactions. The steric and electronic properties of these substituents can direct the approach of reagents, leading to the preferential formation of one diastereomer over others. []
Q11: What is the mechanism of iodination of 3,4,6-Tri-O-benzyl-D-glucal?
A11: Iodination of 3,4,6-Tri-O-benzyl-D-glucal with IN3 in acetonitrile proceeds through a bridged iodonium ion intermediate. This intermediate is attacked by the azide nucleophile from the opposite face, resulting in the formation of trans-α-iodoazides as the major product. The reaction exhibits high diastereoselectivity, regardless of the presence or absence of substituents on the ring. []
Q12: Has computational chemistry been applied to study 3,4,6-Tri-O-benzyl-D-glucal and its derivatives?
A12: While the provided research snippets do not explicitly detail computational studies on 3,4,6-Tri-O-benzyl-D-glucal, computational tools like density functional theory (DFT) calculations and molecular dynamics simulations can be valuable for:
Q13: What insights into Structure-Activity Relationships (SAR) can be derived from modifications to 3,4,6-Tri-O-benzyl-D-glucal?
A13: Modifying the 3,4,6-Tri-O-benzyl-D-glucal structure can provide valuable SAR insights, particularly for applications like glycosidase inhibition. For example:
- Steviamine Analogues: The synthesis and testing of two new steviamine stereoisomers, starting from 3,4,6-Tri-O-benzyl-D-glucal, revealed that the 2,3-di-epi-(-)-steviamine isomer selectively inhibits α-galactosidase. []
- Amino-Modified Iminocyclitols: Exploring various alkylamino substituents on iminocyclitols derived from 3,4,6-Tri-O-benzyl-D-glucal could help identify potent and selective α-galactosidase inhibitors. []
Disclaimer and Information on In-Vitro Research Products
Please be aware that all articles and product information presented on BenchChem are intended solely for informational purposes. The products available for purchase on BenchChem are specifically designed for in-vitro studies, which are conducted outside of living organisms. In-vitro studies, derived from the Latin term "in glass," involve experiments performed in controlled laboratory settings using cells or tissues. It is important to note that these products are not categorized as medicines or drugs, and they have not received approval from the FDA for the prevention, treatment, or cure of any medical condition, ailment, or disease. We must emphasize that any form of bodily introduction of these products into humans or animals is strictly prohibited by law. It is essential to adhere to these guidelines to ensure compliance with legal and ethical standards in research and experimentation.