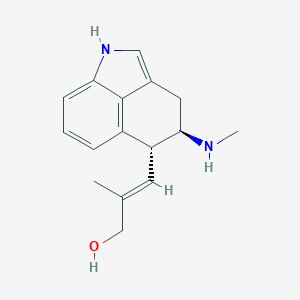
Chanoclavine
Overview
Description
. Ergot alkaloids are known for their complex structures and significant pharmacological effects. Chanoclavine is an important intermediate in the biosynthesis of various ergot alkaloids, which have applications in medicine and agriculture .
Preparation Methods
Synthetic Routes and Reaction Conditions: The biosynthesis of chanoclavine involves several key enzymes. For instance, the combined action of EasC and EasE from Aspergillus japonicus in the yeast Saccharomyces cerevisiae can produce this compound-I . The genomic sequence from an ergot alkaloid producer, Aspergillus japonicus, is used to predict the protein encoding sequences of the early ergot alkaloid pathway genes. These genes are then cloned and expressed in yeast, resulting in the de novo production of this compound-I .
Industrial Production Methods: Industrial production of this compound involves the cultivation of fungi such as Claviceps purpurea. The fungi are grown under controlled conditions to optimize the yield of this compound. The process includes the fermentation of the fungi, followed by extraction and purification of the alkaloid .
Chemical Reactions Analysis
Types of Reactions: Chanoclavine undergoes various chemical reactions, including oxidation, reduction, and substitution. For example, the enzyme EasDaf catalyzes the conversion of this compound-I aldehyde to this compound-I acid through two sequential oxidations . Additionally, this compound-I aldehyde can be converted to festuclavine and pyroclavine through reduction steps .
Common Reagents and Conditions:
Oxidation: Enzymes such as EasDaf and FgaDH are involved in the oxidation of this compound-I to this compound-I acid.
Major Products:
This compound-I acid: Formed through oxidation reactions.
Festuclavine and Pyroclavine: Formed through reduction reactions.
Scientific Research Applications
Chanoclavine has several scientific research applications:
Mechanism of Action
Chanoclavine exerts its effects by interacting with specific molecular targets and pathways. For instance, it has been found to stimulate dopamine D2 receptors in the brain, which is significant for its pharmacological effects . Additionally, this compound interacts with the 5-hydroxytryptamine (5-HT) 3A receptor, regulating conditions such as irritable bowel syndrome . The interaction involves binding to specific amino acid residues in the receptor, leading to competitive inhibition of the receptor’s activity .
Comparison with Similar Compounds
Chanoclavine is part of a group of ergot alkaloids known as ergoclavines. Similar compounds include:
Agroclavine: Another ergot alkaloid with similar biosynthetic pathways.
Elymoclavine: Shares structural similarities with this compound and is also an intermediate in the biosynthesis of other ergot alkaloids.
Uniqueness: this compound is unique due to its specific role as an intermediate in the biosynthesis of various ergot alkaloids. Its ability to interact with multiple molecular targets, such as dopamine receptors and serotonin receptors, highlights its pharmacological significance .
Properties
IUPAC Name |
(E)-2-methyl-3-[(4R,5R)-4-(methylamino)-1,3,4,5-tetrahydrobenzo[cd]indol-5-yl]prop-2-en-1-ol | |
---|---|---|
Source | PubChem | |
URL | https://pubchem.ncbi.nlm.nih.gov | |
Description | Data deposited in or computed by PubChem | |
InChI |
InChI=1S/C16H20N2O/c1-10(9-19)6-13-12-4-3-5-14-16(12)11(8-18-14)7-15(13)17-2/h3-6,8,13,15,17-19H,7,9H2,1-2H3/b10-6+/t13-,15-/m1/s1 | |
Source | PubChem | |
URL | https://pubchem.ncbi.nlm.nih.gov | |
Description | Data deposited in or computed by PubChem | |
InChI Key |
SAHHMCVYMGARBT-HEESEWQSSA-N | |
Source | PubChem | |
URL | https://pubchem.ncbi.nlm.nih.gov | |
Description | Data deposited in or computed by PubChem | |
Canonical SMILES |
CC(=CC1C(CC2=CNC3=CC=CC1=C23)NC)CO | |
Source | PubChem | |
URL | https://pubchem.ncbi.nlm.nih.gov | |
Description | Data deposited in or computed by PubChem | |
Isomeric SMILES |
C/C(=C\[C@H]1[C@@H](CC2=CNC3=CC=CC1=C23)NC)/CO | |
Source | PubChem | |
URL | https://pubchem.ncbi.nlm.nih.gov | |
Description | Data deposited in or computed by PubChem | |
Molecular Formula |
C16H20N2O | |
Source | PubChem | |
URL | https://pubchem.ncbi.nlm.nih.gov | |
Description | Data deposited in or computed by PubChem | |
DSSTOX Substance ID |
DTXSID50893242 | |
Record name | Chanoclavine | |
Source | EPA DSSTox | |
URL | https://comptox.epa.gov/dashboard/DTXSID50893242 | |
Description | DSSTox provides a high quality public chemistry resource for supporting improved predictive toxicology. | |
Molecular Weight |
256.34 g/mol | |
Source | PubChem | |
URL | https://pubchem.ncbi.nlm.nih.gov | |
Description | Data deposited in or computed by PubChem | |
CAS No. |
2390-99-0 | |
Record name | Chanoclavine | |
Source | CAS Common Chemistry | |
URL | https://commonchemistry.cas.org/detail?cas_rn=2390-99-0 | |
Description | CAS Common Chemistry is an open community resource for accessing chemical information. Nearly 500,000 chemical substances from CAS REGISTRY cover areas of community interest, including common and frequently regulated chemicals, and those relevant to high school and undergraduate chemistry classes. This chemical information, curated by our expert scientists, is provided in alignment with our mission as a division of the American Chemical Society. | |
Explanation | The data from CAS Common Chemistry is provided under a CC-BY-NC 4.0 license, unless otherwise stated. | |
Record name | Chanoclavine | |
Source | ChemIDplus | |
URL | https://pubchem.ncbi.nlm.nih.gov/substance/?source=chemidplus&sourceid=0002390990 | |
Description | ChemIDplus is a free, web search system that provides access to the structure and nomenclature authority files used for the identification of chemical substances cited in National Library of Medicine (NLM) databases, including the TOXNET system. | |
Record name | Chanoclavine | |
Source | EPA DSSTox | |
URL | https://comptox.epa.gov/dashboard/DTXSID50893242 | |
Description | DSSTox provides a high quality public chemistry resource for supporting improved predictive toxicology. | |
Record name | CHANOCLAVINE | |
Source | FDA Global Substance Registration System (GSRS) | |
URL | https://gsrs.ncats.nih.gov/ginas/app/beta/substances/32X6F73RE2 | |
Description | The FDA Global Substance Registration System (GSRS) enables the efficient and accurate exchange of information on what substances are in regulated products. Instead of relying on names, which vary across regulatory domains, countries, and regions, the GSRS knowledge base makes it possible for substances to be defined by standardized, scientific descriptions. | |
Explanation | Unless otherwise noted, the contents of the FDA website (www.fda.gov), both text and graphics, are not copyrighted. They are in the public domain and may be republished, reprinted and otherwise used freely by anyone without the need to obtain permission from FDA. Credit to the U.S. Food and Drug Administration as the source is appreciated but not required. | |
Retrosynthesis Analysis
AI-Powered Synthesis Planning: Our tool employs the Template_relevance Pistachio, Template_relevance Bkms_metabolic, Template_relevance Pistachio_ringbreaker, Template_relevance Reaxys, Template_relevance Reaxys_biocatalysis model, leveraging a vast database of chemical reactions to predict feasible synthetic routes.
One-Step Synthesis Focus: Specifically designed for one-step synthesis, it provides concise and direct routes for your target compounds, streamlining the synthesis process.
Accurate Predictions: Utilizing the extensive PISTACHIO, BKMS_METABOLIC, PISTACHIO_RINGBREAKER, REAXYS, REAXYS_BIOCATALYSIS database, our tool offers high-accuracy predictions, reflecting the latest in chemical research and data.
Strategy Settings
Precursor scoring | Relevance Heuristic |
---|---|
Min. plausibility | 0.01 |
Model | Template_relevance |
Template Set | Pistachio/Bkms_metabolic/Pistachio_ringbreaker/Reaxys/Reaxys_biocatalysis |
Top-N result to add to graph | 6 |
Feasible Synthetic Routes
Disclaimer and Information on In-Vitro Research Products
Please be aware that all articles and product information presented on BenchChem are intended solely for informational purposes. The products available for purchase on BenchChem are specifically designed for in-vitro studies, which are conducted outside of living organisms. In-vitro studies, derived from the Latin term "in glass," involve experiments performed in controlled laboratory settings using cells or tissues. It is important to note that these products are not categorized as medicines or drugs, and they have not received approval from the FDA for the prevention, treatment, or cure of any medical condition, ailment, or disease. We must emphasize that any form of bodily introduction of these products into humans or animals is strictly prohibited by law. It is essential to adhere to these guidelines to ensure compliance with legal and ethical standards in research and experimentation.