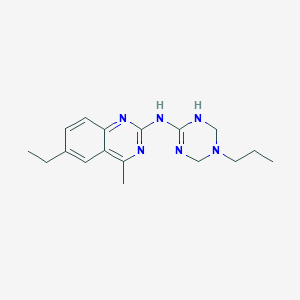
6-ethyl-4-methyl-N-(5-propyl-1,4,5,6-tetrahydro-1,3,5-triazin-2-yl)quinazolin-2-amine
- Click on QUICK INQUIRY to receive a quote from our team of experts.
- With the quality product at a COMPETITIVE price, you can focus more on your research.
Overview
Description
6-ethyl-4-methyl-N-(5-propyl-1,4,5,6-tetrahydro-1,3,5-triazin-2-yl)quinazolin-2-amine is an organic compound with the molecular formula C₁₇H₂₄N₆. This compound is characterized by its complex structure, which includes a quinazoline core, a triazine ring, and various alkyl substituents. It is of interest in various fields of scientific research due to its potential biological and chemical properties .
Preparation Methods
Synthetic Routes and Reaction Conditions
The synthesis of 6-ethyl-4-methyl-N-(5-propyl-1,4,5,6-tetrahydro-1,3,5-triazin-2-yl)quinazolin-2-amine typically involves multi-step organic reactions. The process begins with the preparation of the quinazoline core, followed by the introduction of the triazine ring and the alkyl substituents. Common reagents used in these reactions include alkyl halides, amines, and various catalysts to facilitate the formation of the desired bonds.
Industrial Production Methods
Industrial production methods for this compound would likely involve large-scale synthesis using optimized reaction conditions to maximize yield and purity. This may include the use of continuous flow reactors, high-throughput screening of catalysts, and advanced purification techniques such as chromatography and crystallization.
Chemical Reactions Analysis
Types of Reactions
6-ethyl-4-methyl-N-(5-propyl-1,4,5,6-tetrahydro-1,3,5-triazin-2-yl)quinazolin-2-amine can undergo various types of chemical reactions, including:
Oxidation: The compound can be oxidized to introduce additional functional groups or to modify existing ones.
Reduction: Reduction reactions can be used to alter the oxidation state of the compound, potentially leading to different derivatives.
Substitution: The compound can undergo nucleophilic or electrophilic substitution reactions, where one functional group is replaced by another.
Common Reagents and Conditions
Common reagents used in these reactions include oxidizing agents (e.g., potassium permanganate), reducing agents (e.g., lithium aluminum hydride), and various nucleophiles or electrophiles depending on the desired substitution reaction. Reaction conditions such as temperature, solvent, and pH are carefully controlled to achieve the desired outcome.
Major Products
The major products formed from these reactions depend on the specific reagents and conditions used. For example, oxidation may yield quinazoline derivatives with additional oxygen-containing functional groups, while substitution reactions may introduce new alkyl or aryl groups.
Scientific Research Applications
Antimicrobial Activity
Research has indicated that compounds with similar structures exhibit notable antimicrobial properties. For instance:
- Quinazoline Derivatives : These compounds have shown effectiveness against various bacterial strains. A study highlighted that derivatives of quinazoline possess antibacterial activity comparable to standard antibiotics .
Anticancer Potential
The incorporation of triazine and quinazoline moieties has been linked to anticancer activity:
- Mechanism of Action : Compounds like 6-Ethyl-4-methyl-N-(5-propyl-1,4,5,6-tetrahydro-1,3,5-triazin-2-yl)quinazolin-2-amine may inhibit specific kinases involved in cancer cell proliferation. For example, triazole derivatives have been reported to inhibit the MET kinase with high potency .
Neuroprotective Effects
Preliminary studies suggest that derivatives of quinazoline may offer neuroprotective effects:
- Neurodegenerative Diseases : Compounds similar to this compound are being investigated for their potential to protect against neurodegeneration by modulating neurotransmitter systems .
Pesticidal Activity
The structural characteristics of this compound suggest potential applications in agrochemicals:
- Insecticidal Properties : Research indicates that quinazoline derivatives can act as insecticides due to their ability to disrupt insect hormonal systems .
Polymer Chemistry
The unique chemical structure allows for the incorporation of this compound into polymer matrices:
- Functional Polymers : The presence of nitrogen heterocycles enhances the thermal and mechanical properties of polymers. This could lead to the development of advanced materials with specific functionalities .
Case Studies
Study | Findings | Application |
---|---|---|
Study 1 | Quinazoline derivatives exhibited significant antibacterial activity against MRSA strains (MIC: 0.125–8 μg/mL) | Antimicrobial agents |
Study 2 | Triazole derivatives demonstrated potent inhibition of MET kinase (IC50: 0.24 nM) | Anticancer therapies |
Study 3 | Research on polymer composites showed enhanced thermal stability with the inclusion of quinazoline-based compounds | Material science applications |
Mechanism of Action
The mechanism of action of 6-ethyl-4-methyl-N-(5-propyl-1,4,5,6-tetrahydro-1,3,5-triazin-2-yl)quinazolin-2-amine involves its interaction with specific molecular targets, such as enzymes or receptors. These interactions can modulate various biological pathways, leading to changes in cellular function. The exact molecular targets and pathways involved depend on the specific application and the context in which the compound is used.
Comparison with Similar Compounds
Similar Compounds
Similar compounds to 6-ethyl-4-methyl-N-(5-propyl-1,4,5,6-tetrahydro-1,3,5-triazin-2-yl)quinazolin-2-amine include other quinazoline derivatives and triazine-containing compounds. Examples include:
- 4-methylquinazoline
- 2,4-diamino-6-ethyl-1,3,5-triazine
Uniqueness
What sets this compound apart is its unique combination of structural features, which may confer distinct chemical and biological properties
Biological Activity
6-Ethyl-4-methyl-N-(5-propyl-1,4,5,6-tetrahydro-1,3,5-triazin-2-yl)quinazolin-2-amine is a compound belonging to the class of quinazoline derivatives. Its unique molecular structure incorporates both quinazoline and tetrahydro-triazine moieties, which contribute to its potential biological activities. This article examines the biological activity of this compound, highlighting its pharmacological properties and mechanisms of action.
Chemical Structure and Properties
The compound features a quinazoline core fused with a tetrahydro-1,3,5-triazine structure. The presence of ethyl and propyl groups enhances its stability and solubility. The molecular formula is C16H22N4.
Property | Value |
---|---|
Molecular Formula | C16H22N4 |
Molecular Weight | 270.37 g/mol |
Solubility | Soluble in organic solvents |
Biological Activity Overview
Research indicates that this compound exhibits various biological activities including:
- Anticancer Activity : Studies have shown that compounds with similar structures can inhibit tumorigenesis by modulating enzyme activities involved in cancer cell proliferation and apoptosis .
- Antiviral Properties : The compound's heterocyclic nature suggests potential efficacy against viral infections by interfering with viral replication processes .
- Enzyme Inhibition : It has been observed to interact with specific enzymes or receptors, leading to modulation of their activity.
The biological activity is primarily attributed to the compound's ability to bind to specific molecular targets such as enzymes or receptors involved in disease processes. This binding may lead to:
- Inhibition of Enzyme Activity : Compounds like this can inhibit key enzymes that are crucial for cancer cell survival or viral replication.
- Receptor Modulation : It may act as an agonist or antagonist at certain receptors, influencing signaling pathways critical for cellular functions.
Case Study 1: Anticancer Activity
A study investigating the anticancer properties of quinazoline derivatives highlighted that modifications in the triazine moiety significantly enhanced the inhibitory effects on cancer cell lines. The results indicated that compounds similar to this compound exhibited IC50 values in the micromolar range against various cancer types .
Case Study 2: Antiviral Efficacy
Another research focused on antiviral activity demonstrated that derivatives of triazine compounds showed significant inhibition of viral polymerases. The IC50 values for some derivatives were reported as low as 0.35 μM against specific viral targets .
Comparative Analysis with Similar Compounds
The following table summarizes the biological activities and unique features of related compounds:
Compound Name | Molecular Formula | Biological Activity |
---|---|---|
6-Ethoxy-4-methyl-N-(5-[3-(morpholin-4-yl)propyl]-quinazolin-2-amines | C16H22N4O | Anticancer and antiviral properties |
6-Ethyl-N-(5-(4-methylbenzyl)-triazine derivatives | C16H20N4 | Antiviral activity |
6-Ethyl-N-(5-propyl-triazine derivatives | C15H20N4 | Enzyme inhibition |
Properties
Molecular Formula |
C17H24N6 |
---|---|
Molecular Weight |
312.4 g/mol |
IUPAC Name |
6-ethyl-4-methyl-N-(3-propyl-2,4-dihydro-1H-1,3,5-triazin-6-yl)quinazolin-2-amine |
InChI |
InChI=1S/C17H24N6/c1-4-8-23-10-18-16(19-11-23)22-17-20-12(3)14-9-13(5-2)6-7-15(14)21-17/h6-7,9H,4-5,8,10-11H2,1-3H3,(H2,18,19,20,21,22) |
InChI Key |
GCTZTVIXZOPQCZ-UHFFFAOYSA-N |
Canonical SMILES |
CCCN1CNC(=NC1)NC2=NC(=C3C=C(C=CC3=N2)CC)C |
Origin of Product |
United States |
Disclaimer and Information on In-Vitro Research Products
Please be aware that all articles and product information presented on BenchChem are intended solely for informational purposes. The products available for purchase on BenchChem are specifically designed for in-vitro studies, which are conducted outside of living organisms. In-vitro studies, derived from the Latin term "in glass," involve experiments performed in controlled laboratory settings using cells or tissues. It is important to note that these products are not categorized as medicines or drugs, and they have not received approval from the FDA for the prevention, treatment, or cure of any medical condition, ailment, or disease. We must emphasize that any form of bodily introduction of these products into humans or animals is strictly prohibited by law. It is essential to adhere to these guidelines to ensure compliance with legal and ethical standards in research and experimentation.