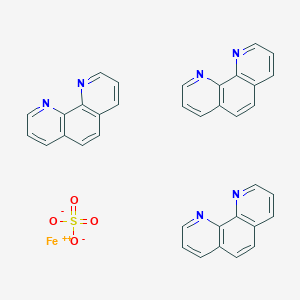
Ferroin
Overview
Description
Ferroin is a chemical compound with the formula [Fe(o-phen)₃]SO₄, where o-phen stands for 1,10-phenanthroline, a bidentate ligand. This compound is widely used as a redox indicator in analytical chemistry due to its distinct color change properties. This compound is known for its vibrant red color in its reduced form and blue color in its oxidized form .
Scientific Research Applications
Mechanism of Action
Target of Action
Ferroin is a chemical compound with the formula [Fe(o-phen)3]SO4, where o-phen is an abbreviation for 1,10-phenanthroline, a bidentate ligand . The primary target of this compound is the redox reactions where it acts as a catalyst. It is used as an indicator in analytical chemistry .
Mode of Action
This compound interacts with its targets through redox reactions. The active ingredient is the [Fe(o-phen)3]2+ ion, which is a chromophore that can be oxidized to the ferric derivative [Fe(o-phen)3]3+ . The main reaction is a 1-electron oxidation .
Biochemical Pathways
This compound affects the redox reactions in biochemical pathways. It is used as a redox indicator in visualizing oscillatory Belousov–Zhabotinsky reactions . The redox potential of the iron-phenanthroline complex can be varied between +0.84 V and +1.10 V by adjusting the position and number of methyl groups on the phenanthroline core .
Pharmacokinetics
It’s known that high oral iron doses or rapid release of iron from intravenous iron preparations can saturate the iron transport system, resulting in oxidative stress with adverse clinical and subclinical consequences .
Result of Action
The result of this compound’s action is the change in color from orange (reduced state) to blue-green (oxidized state), which is used to indicate the progress of redox reactions . This color change is reversible, very pronounced, and rapid .
Action Environment
The action of this compound is influenced by environmental factors such as temperature and pH. This compound is stable up to 60 °C, and its redox potential can be influenced by the acidity of the environment . Furthermore, this compound can be activated by UVA in mildly acidic media in a photo-Fenton-like process .
Safety and Hazards
Ferroin is harmful if swallowed and harmful to aquatic life with long-lasting effects . It is advised to avoid breathing mist, gas, or vapours, and to avoid contact with skin and eyes . Use of personal protective equipment and chemical impermeable gloves is recommended . Ensure adequate ventilation and remove all sources of ignition . In case of a spill or leak, personnel should be evacuated to safe areas .
Biochemical Analysis
Biochemical Properties
Ferroin plays a crucial role in biochemical reactions. It interacts with various enzymes, proteins, and other biomolecules. The nature of these interactions is largely dependent on the oxidation state of the iron in this compound .
Cellular Effects
The effects of this compound on various types of cells and cellular processes are significant. It influences cell function, including impact on cell signaling pathways, gene expression, and cellular metabolism .
Molecular Mechanism
This compound exerts its effects at the molecular level through binding interactions with biomolecules, enzyme inhibition or activation, and changes in gene expression .
Temporal Effects in Laboratory Settings
Over time, the effects of this compound can change in laboratory settings. This includes information on the product’s stability, degradation, and any long-term effects on cellular function observed in in vitro or in vivo studies .
Dosage Effects in Animal Models
The effects of this compound vary with different dosages in animal models. This includes any threshold effects observed in these studies, as well as any toxic or adverse effects at high doses .
Metabolic Pathways
This compound is involved in various metabolic pathways, interacting with enzymes or cofactors. This includes any effects on metabolic flux or metabolite levels .
Transport and Distribution
This compound is transported and distributed within cells and tissues. This includes any transporters or binding proteins that it interacts with, as well as any effects on its localization or accumulation .
Subcellular Localization
The subcellular localization of this compound and any effects on its activity or function include any targeting signals or post-translational modifications that direct it to specific compartments or organelles .
Preparation Methods
Synthetic Routes and Reaction Conditions: Ferroin sulfate can be synthesized by combining 1,10-phenanthroline with ferrous sulfate in water. The reaction is as follows: [ 3 \text{phen} + \text{Fe}^{2+} \rightarrow [\text{Fe(phen)}_3]^{2+} ] This reaction involves the formation of a complex between ferrous ions and 1,10-phenanthroline .
Industrial Production Methods: In industrial settings, the preparation of this compound involves the controlled addition of sulfuric acid to an aqueous solution of the ferrous-phenanthroline complex. This process ensures the stability and purity of the final product .
Types of Reactions:
Oxidation: this compound undergoes a one-electron oxidation reaction, converting from its ferrous (Fe²⁺) form to its ferric (Fe³⁺) form: [ [\text{Fe(phen)}_3]^{2+} \rightarrow [\text{Fe(phen)}_3]^{3+} + e^- ]
Hydrolysis: Addition of sulfuric acid to an aqueous solution of this compound causes hydrolysis: [ [\text{Fe(phen)}_3]^{2+} + 3 \text{H}_2\text{SO}_4 + 6 \text{H}_2\text{O} \rightarrow [\text{Fe(OH}_2)_6]^{2+} + 3 [\text{phenH}]\text{HSO}_4^- ]
Common Reagents and Conditions:
Oxidation: Commonly performed in the presence of sulfuric acid.
Hydrolysis: Requires sulfuric acid and water.
Major Products:
Oxidation: Ferric-phenanthroline complex.
Hydrolysis: Hexaaquairon(II) complex and protonated phenanthroline sulfate.
Comparison with Similar Compounds
Ferroin is often compared with other iron-phenanthroline complexes, such as:
Nitrothis compound: A complex of iron(II) with 5-nitro-1,10-phenanthroline.
Tris(bipyridine)iron(II): Another iron complex with similar redox properties but different ligand structures.
Uniqueness: this compound is unique due to its distinct color change, stability, and versatility as a redox indicator. Its ability to participate in various redox reactions and its application in diverse fields make it a valuable compound in both research and industry .
Properties
IUPAC Name |
iron(2+);1,10-phenanthroline;sulfate | |
---|---|---|
Source | PubChem | |
URL | https://pubchem.ncbi.nlm.nih.gov | |
Description | Data deposited in or computed by PubChem | |
InChI |
InChI=1S/3C12H8N2.Fe.H2O4S/c3*1-3-9-5-6-10-4-2-8-14-12(10)11(9)13-7-1;;1-5(2,3)4/h3*1-8H;;(H2,1,2,3,4)/q;;;+2;/p-2 | |
Source | PubChem | |
URL | https://pubchem.ncbi.nlm.nih.gov | |
Description | Data deposited in or computed by PubChem | |
InChI Key |
CIWXFRVOSDNDJZ-UHFFFAOYSA-L | |
Source | PubChem | |
URL | https://pubchem.ncbi.nlm.nih.gov | |
Description | Data deposited in or computed by PubChem | |
Canonical SMILES |
C1=CC2=C(C3=C(C=CC=N3)C=C2)N=C1.C1=CC2=C(C3=C(C=CC=N3)C=C2)N=C1.C1=CC2=C(C3=C(C=CC=N3)C=C2)N=C1.[O-]S(=O)(=O)[O-].[Fe+2] | |
Source | PubChem | |
URL | https://pubchem.ncbi.nlm.nih.gov | |
Description | Data deposited in or computed by PubChem | |
Molecular Formula |
C36H24FeN6O4S | |
Source | PubChem | |
URL | https://pubchem.ncbi.nlm.nih.gov | |
Description | Data deposited in or computed by PubChem | |
Related CAS |
14708-99-7 (Parent) | |
Record name | 1,10-Phenanthroline ferrous sulfate | |
Source | ChemIDplus | |
URL | https://pubchem.ncbi.nlm.nih.gov/substance/?source=chemidplus&sourceid=0014634914 | |
Description | ChemIDplus is a free, web search system that provides access to the structure and nomenclature authority files used for the identification of chemical substances cited in National Library of Medicine (NLM) databases, including the TOXNET system. | |
DSSTOX Substance ID |
DTXSID8065799 | |
Record name | Iron(2+), tris(1,10-phenanthroline-.kappa.N1,.kappa.N10)-, (OC-6-11)-, sulfate (1:1) | |
Source | EPA DSSTox | |
URL | https://comptox.epa.gov/dashboard/DTXSID8065799 | |
Description | DSSTox provides a high quality public chemistry resource for supporting improved predictive toxicology. | |
Molecular Weight |
692.5 g/mol | |
Source | PubChem | |
URL | https://pubchem.ncbi.nlm.nih.gov | |
Description | Data deposited in or computed by PubChem | |
Physical Description |
Aqueous solution (99.9% water); [Aldrich MSDS] | |
Record name | 1,10-Phenanthroline ferrous sulfate | |
Source | Haz-Map, Information on Hazardous Chemicals and Occupational Diseases | |
URL | https://haz-map.com/Agents/9610 | |
Description | Haz-Map® is an occupational health database designed for health and safety professionals and for consumers seeking information about the adverse effects of workplace exposures to chemical and biological agents. | |
Explanation | Copyright (c) 2022 Haz-Map(R). All rights reserved. Unless otherwise indicated, all materials from Haz-Map are copyrighted by Haz-Map(R). No part of these materials, either text or image may be used for any purpose other than for personal use. Therefore, reproduction, modification, storage in a retrieval system or retransmission, in any form or by any means, electronic, mechanical or otherwise, for reasons other than personal use, is strictly prohibited without prior written permission. | |
CAS No. |
14634-91-4 | |
Record name | Tris(1,10-phenanthroline)iron(II) sulfate | |
Source | CAS Common Chemistry | |
URL | https://commonchemistry.cas.org/detail?cas_rn=14634-91-4 | |
Description | CAS Common Chemistry is an open community resource for accessing chemical information. Nearly 500,000 chemical substances from CAS REGISTRY cover areas of community interest, including common and frequently regulated chemicals, and those relevant to high school and undergraduate chemistry classes. This chemical information, curated by our expert scientists, is provided in alignment with our mission as a division of the American Chemical Society. | |
Explanation | The data from CAS Common Chemistry is provided under a CC-BY-NC 4.0 license, unless otherwise stated. | |
Record name | 1,10-Phenanthroline ferrous sulfate | |
Source | ChemIDplus | |
URL | https://pubchem.ncbi.nlm.nih.gov/substance/?source=chemidplus&sourceid=0014634914 | |
Description | ChemIDplus is a free, web search system that provides access to the structure and nomenclature authority files used for the identification of chemical substances cited in National Library of Medicine (NLM) databases, including the TOXNET system. | |
Record name | Iron(2+), tris(1,10-phenanthroline-.kappa.N1,.kappa.N10)-, (OC-6-11)-, sulfate (1:1) | |
Source | EPA Chemicals under the TSCA | |
URL | https://www.epa.gov/chemicals-under-tsca | |
Description | EPA Chemicals under the Toxic Substances Control Act (TSCA) collection contains information on chemicals and their regulations under TSCA, including non-confidential content from the TSCA Chemical Substance Inventory and Chemical Data Reporting. | |
Record name | Iron(2+), tris(1,10-phenanthroline-.kappa.N1,.kappa.N10)-, (OC-6-11)-, sulfate (1:1) | |
Source | EPA DSSTox | |
URL | https://comptox.epa.gov/dashboard/DTXSID8065799 | |
Description | DSSTox provides a high quality public chemistry resource for supporting improved predictive toxicology. | |
Record name | Tris(1,10-phenanthroline-N1,N10)iron sulphate | |
Source | European Chemicals Agency (ECHA) | |
URL | https://echa.europa.eu/substance-information/-/substanceinfo/100.035.145 | |
Description | The European Chemicals Agency (ECHA) is an agency of the European Union which is the driving force among regulatory authorities in implementing the EU's groundbreaking chemicals legislation for the benefit of human health and the environment as well as for innovation and competitiveness. | |
Explanation | Use of the information, documents and data from the ECHA website is subject to the terms and conditions of this Legal Notice, and subject to other binding limitations provided for under applicable law, the information, documents and data made available on the ECHA website may be reproduced, distributed and/or used, totally or in part, for non-commercial purposes provided that ECHA is acknowledged as the source: "Source: European Chemicals Agency, http://echa.europa.eu/". Such acknowledgement must be included in each copy of the material. ECHA permits and encourages organisations and individuals to create links to the ECHA website under the following cumulative conditions: Links can only be made to webpages that provide a link to the Legal Notice page. | |
Record name | FERROIN SULFATE | |
Source | FDA Global Substance Registration System (GSRS) | |
URL | https://gsrs.ncats.nih.gov/ginas/app/beta/substances/YP88WTW2E4 | |
Description | The FDA Global Substance Registration System (GSRS) enables the efficient and accurate exchange of information on what substances are in regulated products. Instead of relying on names, which vary across regulatory domains, countries, and regions, the GSRS knowledge base makes it possible for substances to be defined by standardized, scientific descriptions. | |
Explanation | Unless otherwise noted, the contents of the FDA website (www.fda.gov), both text and graphics, are not copyrighted. They are in the public domain and may be republished, reprinted and otherwise used freely by anyone without the need to obtain permission from FDA. Credit to the U.S. Food and Drug Administration as the source is appreciated but not required. | |
Retrosynthesis Analysis
AI-Powered Synthesis Planning: Our tool employs the Template_relevance Pistachio, Template_relevance Bkms_metabolic, Template_relevance Pistachio_ringbreaker, Template_relevance Reaxys, Template_relevance Reaxys_biocatalysis model, leveraging a vast database of chemical reactions to predict feasible synthetic routes.
One-Step Synthesis Focus: Specifically designed for one-step synthesis, it provides concise and direct routes for your target compounds, streamlining the synthesis process.
Accurate Predictions: Utilizing the extensive PISTACHIO, BKMS_METABOLIC, PISTACHIO_RINGBREAKER, REAXYS, REAXYS_BIOCATALYSIS database, our tool offers high-accuracy predictions, reflecting the latest in chemical research and data.
Strategy Settings
Precursor scoring | Relevance Heuristic |
---|---|
Min. plausibility | 0.01 |
Model | Template_relevance |
Template Set | Pistachio/Bkms_metabolic/Pistachio_ringbreaker/Reaxys/Reaxys_biocatalysis |
Top-N result to add to graph | 6 |
Feasible Synthetic Routes
Disclaimer and Information on In-Vitro Research Products
Please be aware that all articles and product information presented on BenchChem are intended solely for informational purposes. The products available for purchase on BenchChem are specifically designed for in-vitro studies, which are conducted outside of living organisms. In-vitro studies, derived from the Latin term "in glass," involve experiments performed in controlled laboratory settings using cells or tissues. It is important to note that these products are not categorized as medicines or drugs, and they have not received approval from the FDA for the prevention, treatment, or cure of any medical condition, ailment, or disease. We must emphasize that any form of bodily introduction of these products into humans or animals is strictly prohibited by law. It is essential to adhere to these guidelines to ensure compliance with legal and ethical standards in research and experimentation.