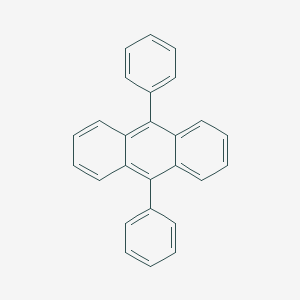
9,10-Diphenylanthracene
Overview
Description
9,10-Diphenylanthracene is a polycyclic aromatic hydrocarbon with the chemical formula C26H18. It is characterized by its slightly yellow powder appearance and is known for its fluorescence properties. This compound is widely used in various scientific and industrial applications due to its unique optical and electronic properties .
Mechanism of Action
Target of Action
9,10-Diphenylanthracene (DPA) is a polycyclic aromatic hydrocarbon . It primarily targets organic light-emitting diodes (OLEDs) and is used as a sensitizer in chemiluminescence . In lightsticks, it is used to produce blue light .
Mode of Action
The mode of action of DPA involves energy transfer mechanisms. Laser photolysis techniques have shown that the quenching of the excited electronic states of DPA involves an energy transfer mechanism resulting in the generation of singlet oxygen from both the lowest singlet and triplet states of the hydrocarbon .
Biochemical Pathways
In dilute solutions, DPA derivatives possess a twisted structure in the ground state that eventually relaxes to a planar structure within picoseconds . The fluorescence process is dominated by the relaxed excited state, and the quantum yield is affected by competition between the nonradiative and radiative deactivations .
Pharmacokinetics
Information on the pharmacokinetics of DPA is limited. It’s known that dpa is a slightly yellow powder and its density is 1.22 g/cm³ . The melting point of DPA is between 248 to 250 °C .
Result of Action
The result of DPA’s action is the production of blue light in lightsticks . It is also useful in blue OLEDs and OLED-based displays . DPA acts as a molecular organic semiconductor .
Action Environment
The action of DPA can be influenced by environmental factors. For instance, the thermal stability of DPA is better in solution-grown crystals than in melt-grown crystals . Furthermore, the near perpendicular orientation of the two phenyl rings at the 9 and 10 position of anthracene causes an elongation in the intermolecular distance of the anthracene cores, which impedes the exciton diffusion rate .
Biochemical Analysis
Biochemical Properties
9,10-Diphenylanthracene is an aromatic hydrocarbon and is a blue light emitting material that is used for the measurement of fluorescence quantum yields in dilute solutions . Its derivatives show potential candidature in organic light emitting diode (OLED) devices . Specific enzymes, proteins, and other biomolecules it interacts with are not mentioned in the available literature.
Temporal Effects in Laboratory Settings
This compound single crystal is a promising scintillator material for fast-neutron detection . The two types of crystals exhibited the same melting point, but the thermal stability of the DPA-Solution crystal is better than that of the DPA-Melt crystal . The long-term effects on cellular function observed in in vitro or in vivo studies are not mentioned in the available literature.
Preparation Methods
Synthetic Routes and Reaction Conditions: 9,10-Diphenylanthracene can be synthesized through a multistep process involving the reaction of 9,10-dibromoanthracene with phenylboronic acid using a tetrakis(triphenylphosphine)palladium(0) catalyst. The reaction is typically carried out under Suzuki coupling conditions . The crude product is then purified by column chromatography to yield the final compound as a light yellow powder .
Industrial Production Methods: In industrial settings, this compound is produced using similar synthetic routes but on a larger scale. The process involves the use of high-purity reagents and optimized reaction conditions to ensure high yield and purity of the final product .
Chemical Reactions Analysis
Types of Reactions: 9,10-Diphenylanthracene undergoes various chemical reactions, including:
Oxidation: It can be oxidized to form anthraquinone derivatives.
Reduction: Reduction reactions can yield dihydro derivatives.
Substitution: Electrophilic substitution reactions can introduce various functional groups onto the anthracene core.
Common Reagents and Conditions:
Oxidation: Common oxidizing agents include potassium permanganate and chromium trioxide.
Reduction: Sodium borohydride and lithium aluminum hydride are frequently used reducing agents.
Substitution: Friedel-Crafts acylation and alkylation reactions are common, using reagents like aluminum chloride and acyl chlorides.
Major Products:
Oxidation: Anthraquinone derivatives.
Reduction: Dihydro derivatives.
Substitution: Various substituted anthracenes depending on the reagents used.
Scientific Research Applications
9,10-Diphenylanthracene has a wide range of applications in scientific research:
Comparison with Similar Compounds
Anthracene: A parent compound with similar fluorescence properties but lower stability.
9,10-Dimethylanthracene: Exhibits higher fluorescence quantum yield but different electronic properties.
2-Chloro-9,10-diphenylanthracene: A chlorinated derivative with altered reactivity and applications
Uniqueness: 9,10-Diphenylanthracene stands out due to its high fluorescence quantum yield, stability, and versatility in various applications, from chemiluminescence to OLEDs .
Properties
IUPAC Name |
9,10-diphenylanthracene | |
---|---|---|
Source | PubChem | |
URL | https://pubchem.ncbi.nlm.nih.gov | |
Description | Data deposited in or computed by PubChem | |
InChI |
InChI=1S/C26H18/c1-3-11-19(12-4-1)25-21-15-7-9-17-23(21)26(20-13-5-2-6-14-20)24-18-10-8-16-22(24)25/h1-18H | |
Source | PubChem | |
URL | https://pubchem.ncbi.nlm.nih.gov | |
Description | Data deposited in or computed by PubChem | |
InChI Key |
FCNCGHJSNVOIKE-UHFFFAOYSA-N | |
Source | PubChem | |
URL | https://pubchem.ncbi.nlm.nih.gov | |
Description | Data deposited in or computed by PubChem | |
Canonical SMILES |
C1=CC=C(C=C1)C2=C3C=CC=CC3=C(C4=CC=CC=C42)C5=CC=CC=C5 | |
Source | PubChem | |
URL | https://pubchem.ncbi.nlm.nih.gov | |
Description | Data deposited in or computed by PubChem | |
Molecular Formula |
C26H18 | |
Source | PubChem | |
URL | https://pubchem.ncbi.nlm.nih.gov | |
Description | Data deposited in or computed by PubChem | |
DSSTOX Substance ID |
DTXSID1061734 | |
Record name | Anthracene, 9,10-diphenyl- | |
Source | EPA DSSTox | |
URL | https://comptox.epa.gov/dashboard/DTXSID1061734 | |
Description | DSSTox provides a high quality public chemistry resource for supporting improved predictive toxicology. | |
Molecular Weight |
330.4 g/mol | |
Source | PubChem | |
URL | https://pubchem.ncbi.nlm.nih.gov | |
Description | Data deposited in or computed by PubChem | |
Physical Description |
Yellow or tan powder; [Alfa Aesar MSDS] | |
Record name | 9,10-Diphenylanthracene | |
Source | Haz-Map, Information on Hazardous Chemicals and Occupational Diseases | |
URL | https://haz-map.com/Agents/11328 | |
Description | Haz-Map® is an occupational health database designed for health and safety professionals and for consumers seeking information about the adverse effects of workplace exposures to chemical and biological agents. | |
Explanation | Copyright (c) 2022 Haz-Map(R). All rights reserved. Unless otherwise indicated, all materials from Haz-Map are copyrighted by Haz-Map(R). No part of these materials, either text or image may be used for any purpose other than for personal use. Therefore, reproduction, modification, storage in a retrieval system or retransmission, in any form or by any means, electronic, mechanical or otherwise, for reasons other than personal use, is strictly prohibited without prior written permission. | |
CAS No. |
1499-10-1 | |
Record name | 9,10-Diphenylanthracene | |
Source | CAS Common Chemistry | |
URL | https://commonchemistry.cas.org/detail?cas_rn=1499-10-1 | |
Description | CAS Common Chemistry is an open community resource for accessing chemical information. Nearly 500,000 chemical substances from CAS REGISTRY cover areas of community interest, including common and frequently regulated chemicals, and those relevant to high school and undergraduate chemistry classes. This chemical information, curated by our expert scientists, is provided in alignment with our mission as a division of the American Chemical Society. | |
Explanation | The data from CAS Common Chemistry is provided under a CC-BY-NC 4.0 license, unless otherwise stated. | |
Record name | 9,10-Diphenylanthracene | |
Source | ChemIDplus | |
URL | https://pubchem.ncbi.nlm.nih.gov/substance/?source=chemidplus&sourceid=0001499101 | |
Description | ChemIDplus is a free, web search system that provides access to the structure and nomenclature authority files used for the identification of chemical substances cited in National Library of Medicine (NLM) databases, including the TOXNET system. | |
Record name | 9,10-DIPHENYLANTHRACENE | |
Source | DTP/NCI | |
URL | https://dtp.cancer.gov/dtpstandard/servlet/dwindex?searchtype=NSC&outputformat=html&searchlist=24861 | |
Description | The NCI Development Therapeutics Program (DTP) provides services and resources to the academic and private-sector research communities worldwide to facilitate the discovery and development of new cancer therapeutic agents. | |
Explanation | Unless otherwise indicated, all text within NCI products is free of copyright and may be reused without our permission. Credit the National Cancer Institute as the source. | |
Record name | Anthracene, 9,10-diphenyl- | |
Source | EPA Chemicals under the TSCA | |
URL | https://www.epa.gov/chemicals-under-tsca | |
Description | EPA Chemicals under the Toxic Substances Control Act (TSCA) collection contains information on chemicals and their regulations under TSCA, including non-confidential content from the TSCA Chemical Substance Inventory and Chemical Data Reporting. | |
Record name | Anthracene, 9,10-diphenyl- | |
Source | EPA DSSTox | |
URL | https://comptox.epa.gov/dashboard/DTXSID1061734 | |
Description | DSSTox provides a high quality public chemistry resource for supporting improved predictive toxicology. | |
Record name | 9,10-diphenylanthracene | |
Source | European Chemicals Agency (ECHA) | |
URL | https://echa.europa.eu/substance-information/-/substanceinfo/100.014.641 | |
Description | The European Chemicals Agency (ECHA) is an agency of the European Union which is the driving force among regulatory authorities in implementing the EU's groundbreaking chemicals legislation for the benefit of human health and the environment as well as for innovation and competitiveness. | |
Explanation | Use of the information, documents and data from the ECHA website is subject to the terms and conditions of this Legal Notice, and subject to other binding limitations provided for under applicable law, the information, documents and data made available on the ECHA website may be reproduced, distributed and/or used, totally or in part, for non-commercial purposes provided that ECHA is acknowledged as the source: "Source: European Chemicals Agency, http://echa.europa.eu/". Such acknowledgement must be included in each copy of the material. ECHA permits and encourages organisations and individuals to create links to the ECHA website under the following cumulative conditions: Links can only be made to webpages that provide a link to the Legal Notice page. | |
Record name | 9,10-DIPHENYLANTHRACENE | |
Source | FDA Global Substance Registration System (GSRS) | |
URL | https://gsrs.ncats.nih.gov/ginas/app/beta/substances/51BQ8IYQ9U | |
Description | The FDA Global Substance Registration System (GSRS) enables the efficient and accurate exchange of information on what substances are in regulated products. Instead of relying on names, which vary across regulatory domains, countries, and regions, the GSRS knowledge base makes it possible for substances to be defined by standardized, scientific descriptions. | |
Explanation | Unless otherwise noted, the contents of the FDA website (www.fda.gov), both text and graphics, are not copyrighted. They are in the public domain and may be republished, reprinted and otherwise used freely by anyone without the need to obtain permission from FDA. Credit to the U.S. Food and Drug Administration as the source is appreciated but not required. | |
Synthesis routes and methods I
Procedure details
Synthesis routes and methods II
Procedure details
Retrosynthesis Analysis
AI-Powered Synthesis Planning: Our tool employs the Template_relevance Pistachio, Template_relevance Bkms_metabolic, Template_relevance Pistachio_ringbreaker, Template_relevance Reaxys, Template_relevance Reaxys_biocatalysis model, leveraging a vast database of chemical reactions to predict feasible synthetic routes.
One-Step Synthesis Focus: Specifically designed for one-step synthesis, it provides concise and direct routes for your target compounds, streamlining the synthesis process.
Accurate Predictions: Utilizing the extensive PISTACHIO, BKMS_METABOLIC, PISTACHIO_RINGBREAKER, REAXYS, REAXYS_BIOCATALYSIS database, our tool offers high-accuracy predictions, reflecting the latest in chemical research and data.
Strategy Settings
Precursor scoring | Relevance Heuristic |
---|---|
Min. plausibility | 0.01 |
Model | Template_relevance |
Template Set | Pistachio/Bkms_metabolic/Pistachio_ringbreaker/Reaxys/Reaxys_biocatalysis |
Top-N result to add to graph | 6 |
Feasible Synthetic Routes
Q1: What is the molecular formula and weight of 9,10-Diphenylanthracene?
A1: this compound has the molecular formula C26H18 and a molecular weight of 330.42 g/mol.
Q2: What spectroscopic data is available for DPA?
A2: DPA exhibits characteristic absorption and emission spectra. Its absorption spectrum lies in the UV region, with peaks between 345 and 420 nm. [] Upon excitation, DPA displays strong blue fluorescence, with emission peaks observed in the range of 400 to 550 nm. [] Furthermore, time-resolved Fourier transform Raman spectroscopy has been utilized to study the excited singlet state of DPA. []
Q3: How does the morphology of DPA crystals affect their performance in optoelectronic applications?
A3: The morphology of DPA crystals significantly influences their optical and electronic properties. For instance, controlling the crystallization process using solution additives can yield different polymorphs of DPA, each with distinct properties. [] Additionally, DPA nanoaggregates and thin films exhibit distinct emission properties compared to their solution-state counterparts. [] Shape-controlled DPA micro/nanostructures, such as octahedra, spheres, microrods, and nanowires, have also been fabricated and incorporated into light-emitting devices, demonstrating the impact of morphology on device performance. []
Q4: Can DPA be used in aqueous environments?
A5: While DPA itself has limited solubility in water, researchers have developed strategies to overcome this limitation. For instance, porous SiO2 nanoparticles containing DPA can be dispersed in water, forming a stable colloidal suspension that retains the characteristic photoluminescence of DPA. []
Q5: Does DPA exhibit any catalytic properties?
A6: While DPA is not typically used as a catalyst itself, it has been investigated as a model compound in catalytic hydrogenation reactions. Studies have explored the mechanism of Fe- or Ni-catalyzed hydrogenation of DPA in the presence of sulfur, revealing that the presence of sulfur significantly influences the reaction pathway. []
Q6: Have computational methods been used to study DPA and its derivatives?
A7: Yes, computational chemistry has played a significant role in understanding the properties and behavior of DPA. Theoretical studies have investigated the triplet-triplet annihilation (TTA) process of DPA in solution, providing insights into the mechanism and kinetics of this phenomenon. [] Furthermore, density functional theory (DFT) calculations have been employed to explore the mechanism of photochemical oxidation involving a uranium(IV) complex containing a DPA derivative, revealing the formation of a fleeting uranium cis-dioxo intermediate. []
Q7: How do structural modifications of DPA affect its properties?
A8: Modifying the DPA structure can significantly impact its photophysical and electrochemical properties. For example, introducing nitrogen atoms into the DPA framework, such as in 2-aza-9,10-diphenylanthracene, alters its electrochemical and optical properties and affects its performance in organic light-emitting diodes (OLEDs). [] Attaching bulky alkyl chains to DPA, as in Cn-sDPAs, can enhance the electron transfer of TTA through the overlap of pseudo-π-orbitals extended to the alkyl chains. []
Q8: What analytical techniques are commonly used to characterize DPA?
A8: Various analytical techniques are employed to characterize DPA and its derivatives. These include:
- Spectroscopy: UV-Vis absorption and fluorescence spectroscopy are essential for analyzing the optical properties of DPA. [, ]
- Microscopy: Field emission scanning electron microscopy (FESEM) and transmission electron microscopy (TEM) are used to visualize the morphology of DPA micro/nanostructures. []
- X-ray Diffraction (XRD): This technique provides insights into the crystal structure and crystallinity of DPA. [, ]
- Electrochemistry: Cyclic voltammetry is used to study the electrochemical behavior of DPA and its derivatives. [, , ]
- Chromatography: Gas chromatography-mass spectrometry (GC-MS) is employed to analyze the products of DPA hydrogenation reactions. []
Q9: What are the main applications of DPA?
A9: DPA's unique properties lend themselves to various applications, including:
- Optoelectronics: DPA is employed as an active material in OLEDs [, ], organic solar cells, and as a blue emitter in light-emitting devices. []
- Scintillators: DPA crystals have shown potential as scintillators due to their relatively high light yield and good pulse shape discrimination properties. []
- Sensors: DPA-based systems have been explored for sensing applications, including the development of an ECL immunosensor for ultrasensitive detection of Aflatoxin B1 using DPA cubic nanoparticles. []
- Photocatalysis: Supramolecular photocatalysts based on DPA have been studied for the synthesis of gold nanocrystals. []
- Photon Upconversion: DPA derivatives are investigated for their potential in triplet fusion upconversion (TF-UC) applications, particularly in solid-state systems. [, ]
- Bioimaging: Nanoscale metal-organic frameworks (MOFs) based on DPA derivatives exhibit two-photon-excited fluorescence, suggesting potential applications in bioimaging. []
Disclaimer and Information on In-Vitro Research Products
Please be aware that all articles and product information presented on BenchChem are intended solely for informational purposes. The products available for purchase on BenchChem are specifically designed for in-vitro studies, which are conducted outside of living organisms. In-vitro studies, derived from the Latin term "in glass," involve experiments performed in controlled laboratory settings using cells or tissues. It is important to note that these products are not categorized as medicines or drugs, and they have not received approval from the FDA for the prevention, treatment, or cure of any medical condition, ailment, or disease. We must emphasize that any form of bodily introduction of these products into humans or animals is strictly prohibited by law. It is essential to adhere to these guidelines to ensure compliance with legal and ethical standards in research and experimentation.